the Creative Commons Attribution 4.0 License.
the Creative Commons Attribution 4.0 License.
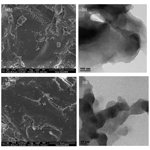
Adsorption and desorption studies of Delonix regia pods and leaves: removal and recovery of Ni(II) and Cu(II) ions from aqueous solution
Bolanle M. Babalola
Adegoke O. Babalola
Cecilia O. Akintayo
Olayide S. Lawal
Sunday F. Abimbade
Ekemena O. Oseghe
Lukman S. Akinola
Olushola S. Ayanda
In this study, the adsorption of Ni(II) and Cu(II) ions from aqueous solutions by powdered Delonix regia pods and leaves was investigated using batch adsorption techniques. The effects of operating conditions such as pH, contact time, adsorbent dosage, metal ion concentration and the presence of sodium ions interfering with the sorption process were investigated. The results obtained showed that equilibrium sorption was attained within 30 min of interaction, and an increase in the initial concentration of the adsorbate, pH and adsorbent dosage led to an increase in the amount of Ni(II) and Cu(II) ions adsorbed. The adsorption process followed the pseudo-second-order kinetic model for all metal ions' sorption. The equilibrium data fitted well with both the Langmuir and Freundlich isotherms; the monolayer adsorption capacity (Q0 mg g−1) of the Delonix regia pods and leaves was 5.88 and 5.77 mg g−1 for Ni(II) ions respectively and 9.12 and 9.01 mg g−1 for Cu(II) ions respectively. The efficiency of the powdered pods and leaves of Delonix regia with respect to the removal of Ni(II) and Cu(II) ions was greater than 80 %, except for the sorption of Ni(II) ions onto the leaves. The desorption study revealed that the percentage of metal ions recovered from the pods was higher than that recovered from the leaves at various nitric acid concentrations. This study proves that Delonix regia biomass, an agricultural waste product (“agro-waste”), could be used to remove Ni(II) and Cu(II) ions from aqueous solution.
Their persistent nature, non-biodegradability, toxicity and ability to bioaccumulate in the environment have made heavy metals priority pollutants (Hamza et al., 2013). Various health effects are caused by anthropogenic pollutants, which are mainly comprised of heavy metals such as mercury, nickel, lead, cadmium copper, zinc and cobalt, in water (Hamza et al., 2013; Singh et al., 2011). Heavy metals gain entrance into water resources via industrial activities such as electroplating; smelting; the production of glass, textile, paper and ceramics; mining; storage batteries; petroleum; metal finishing; pulp and paper (Dean et al., 1972; Ksakas et al., 2018; Kumar et al., 2019). The damage caused by copper to marine life includes damage to the gills, liver, nervous system and kidneys as well as an alteration of the sexual life of fishes (Flemming and Trevors, 1989; Ho et al., 2002; Van Genderen et al., 2005). Although, copper is known to play a vital role in metabolism in animals, its excessive intake can result in serious health problems (Paulino et al., 2006). The permissible limit of copper in drinking water and wastewater is 0.5 and 2.5 mg L−1 respectively (Zhou et al., 2018; Kumar et al., 2019). Reactive free oxygen species that damage lipids, proteins and DNA are released when copper is present in the blood system (Brewer, 2010). Wilson's and Alzheimer's diseases, mental illness, Indian childhood cirrhosis and schizophrenia are also reported to be caused by excess copper in the blood (Brewer, 2007; Faller, 2009; Hurean and Faller, 2009). Nickel has detrimental effects on human health, resulting in allergic dermatitis, immunologic urticaria, and immediate and delayed hypersensitivity (Festus et al., 2013). All nickel compounds, except for metallic nickel, have been classified as human carcinogens by the International Agency for Research on Cancer (IARC, 1990) and the U.S. Department of Health and Human Services (DHHS, 1994). Due to its toxicity in minute quantities, the permissible limit of nickel in wastewater has been set at 0.05 mg L−1 (Zhou et al., 2018).
Conventional methods for the removal of metal ions include the following: chemical precipitation, oxidation/reduction, ion exchange, electrochemical processes, membrane separation, the Fenton process, ozonization, electrocoagulation, photochemical degradation and evaporation (Okoya et al., 2014). These techniques have high operational costs and yield minimal removal efficiencies; hence, they have been reported to be expensive and inadequate (e.g. Okoya et al., 2014). Therefore, there is the need to investigate alternative techniques that are cheaper, efficient and easy to handle. One such technique is biosorption, which involves the use of low-cost adsorbents like agricultural materials of no economic value and industrial by-products (Jeme, 1968; Inoue and Munemori, 1979) for the removal of heavy metal ions from polluted water.
Almond shells and tree bark treated with formaldehyde and sulfuric acid (Guibal et al., 1993; Raji et al., 1997), bone char, tea leaves, wood charcoal (Ajmal et al., 2003) and coconut shells have all been used to produce activated carbon to remove heavy metal ions from wastewater. Rice hulls (Ajmal et al., 2003), rice bran (Montanher et al., 2005) and pine bark (Nath et al., 1997) have also been used in their raw and treated forms to remove heavy metal ions. The removal of Ni(II) ions from aqueous solution using sugarcane bagasse, an agricultural waste biomass, was investigated by Garg et al. (2008). The adsorbent dosage for the maximum removal of Ni(II) ions from a 50 mg L−1 aqueous solution was reported to be 1500 mg L−1 at a pH of 7.5. Moodley et al. (2011) investigated the adsorption capacity of pine sawdust by treating wastewater containing Ni(II) and other metal ions (Co(II) and Fe(III) ions). The adsorption and desorption of Ni(II) ions from aqueous solution by a lignocellulose/montmorillonite nanocomposite was reported by Zhang and Wang (2015). Their report indicated that the maximum adsorption capacity of Ni(II) ions reached 94.86 mg g−1 at an initial Ni(II) ion concentration of 0.0032 mol L−1, a solution pH of 6.8, a temperature of 70 ∘C and a contact time of 40 min. Kahraman et al. (2008) examined the use of cotton stalk and apricot seeds as alternative adsorbents for the removal of Pb and Cu. The removal of Pb and Cu using these agricultural waste products was reported to reduce their toxic effects on Pseudomonas aeruginosa. The sorption capacity of Cu(II) as well as the kinetics and isotherms of different low-cost residual agricultural materials including peanut shells, nut shells, plum seeds, eucalyptus bark, olive pips, peach stones and pine sawdust was studied by Hansen et al. (2010). Moreover, Abdel-Tawwab et al. (2017) used rice straw, sugarcane bagasse and maize stalks for the removal of Pb, Cd, Cu and Zn from aqueous solution. All of the biosorbents were reported to be effective and cheap for the removal of the above-mentioned metal ions from polluted water, with rice straw showing a higher adsorption efficiency than the others. The application of treated pumpkin husk as an excellent adsorbent for removing Cu(II) and Ni(II) ions was reported by Samuel et al. (2016). The adsorption of Cu(II) and Ni(II) ions was found to be suitable at a pH of 5.
Delonix regia , also known as flame of the forest, is a semi-deciduous tree that is native to Madagascar. It is popularly grown as a shade tree and for ornamental purposes in Africa and Hong Kong. The tree has pods that can be as long as 60 cm in length and 5 cm wide as well as distinctive bright green fern-like compound leaves and attractive red peacock flowers. Researchers have reported the usefulness of the green leaves and flowers in medicine: Delonix regia has a broad spectrum of pharmacological activities for various ailments (Modi et al., 2016). However, the tree sheds its leaves and flowers in dry areas and during dry seasons, and the trees are less attractive after the leaves and flowers are shed, with their pods remaining on the branches until they are dropped by wind; this makes Delonix regia an agro-waste with limited valuable use.
There are limited studies on the use of Delonix regia for the removal of organic and inorganic contaminants from aqueous solution, except for Ponnusami et al. (2009), Onwuka et al. (2016) and Babalola et al. (2019) who reported the viability of Delonix regia for the removal of methylene blue dye, a crude oil spill and Pb(II) ions respectively. Therefore, the objective of this research is to investigate the capacity of Delonix regia pods and leaves to remove Ni(II) and Cu(II) ions from aqueous solutions. The desorption of bound metals from spent Delonix regia pods and leaves using various concentrations of nitric acid is also considered.
2.1 Delonix regia sample
Delonix regia leaves and pods collected from Ekiti State University, Ado-Ekiti, Nigeria, were used as the adsorbent for the sorption study. The materials were washed with deionized water, sun-dried and milled. After milling, the adsorbents were sieved through a 250 µm mesh nylon sieve and kept in air-tight containers until required for use.
2.2 Chemicals and reagents
Diammonium nickel hexahydrate and copper chloride dihydrate salts supplied by Merck, Germany, were dissolved in high-purity Milli-Q water to prepare 1000 mg L−1 stock solutions of Ni(II) and Cu(II) ions respectively. Working standard solutions were prepared from the stock solutions, and pH adjustment was carried out with 0.1 M HNO3 and 0.1 M NaOH when necessary. The effect of the solutions' ionic strength on sorption was studied using different concentrations of sodium nitrate salt, and the desorption of bound metal from spent biomass was achieved using different concentrations of HNO3.
2.3 Characterization
The elemental composition of the Delonix regia pods and leaves was established using energy dispersive spectroscopy (EDS). The morphological study was undertaken using a scanning electron microscope (SEM; Nova NanoSEM 230) and a transmission electron microscope (TEM; FEI Tecnai G2 20). An X-ray diffractometer (Siemens D8 ADVANCE Bruker XRD) was used for the phase characterization.
2.4 Adsorption
The adsorption procedure from Meena et al. (2008) was slightly modified and was used in this work. Parameters such as the influence of pH, contact time, initial adsorbate concentration, adsorbent dosage and the solutions' ionic strength were investigated.
To investigate the influence of pH, 0.5 g of biomass was weighed into respective designated tubes containing 20 mL of 100 mg L−1 metal ion solution with various pH levels ranging from 1 to 8. The suspensions were shaken on an end-over-end shaker for 300 min at ambient temperature (21±2 ∘C). The influence of contact time was explored by varying the contact time from 5 to 300 min at optimum pH. The concentration of Ni(II) and Cu(II) ions was varied from 1 to 1000 mg L−1 to investigate the influence of the initial metal ion concentration at optimum pH and contact time. Finally, the influence of the adsorbent dosage and the solution's ionic strength were explored using a 100 mg L−1 metal ion concentration, and the parameters were varied from 0.25 to 1.0 g and from 0.001 to 0.5 M NaNO3 respectively at optimum pH and contact time. Each of the experiments was carried out in triplicate, and the results presented are the average values.
At the expiration of each of the experiment, an aliquot was taken, centrifuged (twice at 4500 rpm for 15 min and once at 10300 rpm for 10 min) and diluted with 0.1 M HNO3 before the residual metal content was analysed by inductively coupled plasma mass spectroscopy (ICP-MS; Thermo Elemental X Series II).
2.5 Analytical procedure
Multi-element standard solution was used to prepare the different concentrations of external calibration standard solutions (1–100 µg L−1) that were used for the analysis. Once the instrument was operational, the pressure of the nebulizer was checked, and the instrument's sensitivity and stability were checked by running a tuning solution of 1 µg L−1 multi-element standard solution for 10 min. It was ensured that the 115In counts were less than 20 K counts per second with a precision of less than 2 %. The backgrounds (backgrounds 5 and 220) were less than 1 count per second, the oxides cerium oxide (CeO+) was less than 2.0 % and the doubly charged ions (Ba2+) were less than 6.0 %. Therefore, the calibration standard solution and the blank were analysed to obtain a calibration curve where the samples to be analysed would fit properly. The analysis of the samples proceeded after the calibration curve for each of the metal ions was suitable (R2=0.9999).
The amount of Ni(II) and Cu(II) ions sorbed on the Delonix regia pods and leaves (Qe) was calculated using Eq. (1):
where Co is the initial metal concentration (mg L−1); Ce is the final metal ion concentration in the solution, obtained from the ICP-MS reading (mg L−1); V is the volume of the metal solution used in litres (L); and m is the mass of the biosorbent (g).
2.6 Kinetics and equilibrium modelling
Further examination of the contact time experiment was carried out by modelling the data with the pseudo-second-order kinetic model to determine the mechanism of the sorption process.
The pseudo-second-order kinetic model can be expressed using Eq. (2), where k2 (g mg−1 min−1) represents the rate constant for the pseudo-second-order kinetics, and Qe and Qt (both in mg g−1) represent the amount of adsorbate taken up by the adsorbent at equilibrium and at time t respectively. All parameters in Eq. (2) were derived by plotting t∕Qt against t.
The data obtained on the effect of the initial metal ion concentration were also modelled using the Langmuir and Freundlich isotherms. A monolayer surface coverage, the availability of an equal number of adsorption sites on the adsorbent and no interaction between the adsorbed species were assumptions of the Langmuir model (Liu and Wang, 2014). The Langmuir isotherm is represented using Eq. (3):
where Qe (mg g−1) is the amount of solute adsorbed per unit mass of adsorbent, Ce (mg L−1) is the equilibrium concentration of the solute in the bulk solution, Q0 (mg g−1) represents the monolayer adsorption capacity of the adsorbent and b (L mg−1) represents the constant related to the energy of adsorption. The Langmuir equation could be further expressed using the dimensionless constant separation factor KR shown in Eq. (4). KR is a relationship containing all of the essential features of the Langmuir isotherm:
where Ci is the initial concentration of metal ions in solution (mg L−1), and Ka represents the Langmuir constant (L mg−1). This dimensionless separation factor is interpreted to imply that the isotherm is favourable if 0<KR<1.
The Freundlich isotherm states that the ratio of the amount of solute adsorbed onto a given mass of the adsorbent to the concentration of the solute in the solution is not constant at different concentrations. The Freundlich isotherm is used to describe adsorption onto heterogeneous surfaces:
where 1∕n is the constant related to the adsorption efficiency, KF is the adsorption capacity, Qe is the quantity of adsorbate adsorbed per unit weight of the adsorbent and Ce is the final concentration of metal in the solution. The Freundlich constant 1∕n is a factor that gives an indication of how favourable the adsorption of the adsorbate onto the sorbent is: 0<1∕n<1 implies favourable adsorption. It is also related to the heterogeneity of the adsorbent surface.
3.1 SEM-EDS, TEM and XRD analyses
The SEM (at ×10 000 magnification) and TEM micrographs of the Delonix regia pods and leaves (Fig. 1) indicated the spongy nature of Delonix regia, with the porous structures being potentially beneficial for the uptake of Ni(II) and Cu(II) ions from aqueous solution. The EDS of Delonix regia showed that the surfaces of the leaves were composed of 66.79 % C, 32.97 % O and traces of Ca and K, whereas the pods were composed of 57.61 % C, 41.15 % O and traces of K.
According to the ICSD (Inorganic Crystal Structure Database) nos. 03-0289, 20-0231 and 26-1077, native cellulose ((C6H12O6)x) and whewellite (C2CaO4•H2O∕CaC2O4•H2O) were identified as major peaks in Delonix regia (Fig. 3). The pods were mainly native cellulose, whereas the leaves were rich in whewellite. The hemicellulosic moieties in the pods and leaves might provide sites for the binding of Ni(II) and Cu(II) ions.
3.2 Adsorption studies
3.2.1 pH
The results regarding the effect of pH on the adsorption of Ni(II) and Cu(II) ions onto Delonix regia are shown in Fig. 4. The figure reveals that there was no evident nickel uptake by either of the adsorbents at low pH, but there was a very noticeable uptake achieved as the pH increased. A pH of 5, at which maximum sorption was recorded, was used in subsequent experiments. Saeed et al. (2005b) reported an optimum sorption pH of 6 for Ni(II) on crop milling waste, whereas a pH of 5 was reported for its sorption onto natural neem sawdust and almond husk (Hasar, 2003; Rao et al., 2007); these values are in line with our findings.
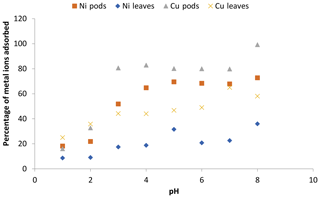
Figure 4The effect of pH on the adsorption of Ni(II) and Cu(II) by Delonix regia pods and leaves. The experimental conditions were as follows: a pH from 1 to 8, a contact time of 300 min, an adsorbent dosage of 0.5 and a metal ion concentration of 100 mg L−1.
A small increase in the uptake value was achieved between a pH of 1 and 3. However, a maximum uptake of 46.7 % was recorded at a pH of 5 for Cu(II) ion sorption onto leaves, and a maximum uptake of 82.9 % was noted at a pH of 4 for Cu(II) ion sorption onto pods; these values were further used for the adsorption of Cu(II) onto leaves and pods respectively. The increase recorded at pH values of 6 and 7 was not considered when choosing the optimum pH at which Cu(II) ion sorption onto leaves occurred due to the presence of visible precipitate which might infer the formation of Cu(OH)2 or other soluble complexes in the experimental set-up at those pH levels (Larous and Meniai, 2012). Some authors have reported pH values of 5 and 5.8 as optimum for the uptake of Cu(II) ions by some modified natural wastes (Shukla and Pai, 2005; Witek-Krowiak et al., 2011; Anantha and Kota, 2016). This might be due to the increased overall negative charge, particularly between a pH of 4 and 6, which would subsequently lead to an increase in the sorption of positive metal ions. An increase in the pH decreases the concentration of hydrogen ions; therefore, competition between metal ions and hydrogen ions for active sites on the adsorbent is reduced (Kadirvelu et al., 2000, 2001, 2003; Sánchez-Polo and Rivera-Utrilla, 2002; Meena et al., 2005a).
3.2.2 Contact time
The investigation of the effect of agitation time, performed at different time intervals from 5 min up to 300 min, showed that the two adsorbents used in this work had a rapid uptake of the metal ions within a short period of interaction (Fig. 5a).
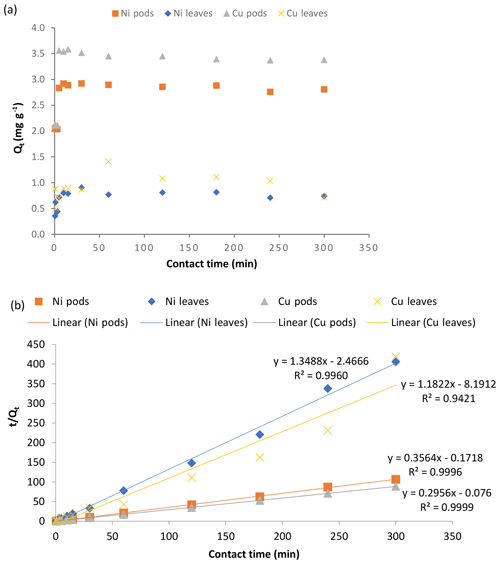
Figure 5Effect of contact time (a) and pseudo-second-order kinetics (b) on the adsorption of Ni(II) and Cu(II) ions by Delonix regia pods and leaves. The experimental conditions were as follows: a pH of 4 and 5, a contact time of 5–300 min, an adsorbent dosage of 0.5 g and a metal ion concentration of 100 mg L−1.
A slight desorption and adsorption was observed after 5 to 10 min of adsorbent interaction with the metal ion solutions. Within 30 min of interaction, maximum uptake had been achieved for both adsorbents, irrespective of the metal ions considered, although the uptake recorded on the pods was higher than that for the leaves. Native cellulose, a major component of the pods might be responsible for the enhanced uptake on the pods. Metal uptake by the adsorbents remained fairly constant after 30 min of interaction until the end of the experiment. Moreover, the maximum uptake at 30 min could be considered to be faster when compared with other biosorbents reported in the literature. Olufemi and Eniodunmo (2018) reported 30 and 120 min as the optimum time for the removal of Ni(II) ions from aqueous solutions using coconut shell and banana peels respectively. Ksakas et al. (2018) reported 60 and 180 min as the optimum contact time for the sorption of Cu(II) ions onto some types of natural clay. Alatabe (2018) reported 120 min as the optimum sorption time for Cu(II) ions onto activated carbon from Cane papyrus. However, 30 min was the optimum contact time reported by Kumar et al. (2019) for the removal of Cu(II) ions using groundnut seed cake powder, sesame seed cake powder and coconut cake powder.
The kinetic modelling of data showed that the process was well fitted to the pseudo-second-order kinetic model. This is supported by Hansen et al. (2010), who reported that the adsorption of Cu(II) ions onto various agricultural waste materials fitted the pseudo-second-order kinetic model. The plots and kinetic parameters of this model are shown in Fig. 5b and Table 1 respectively. The pseudo-second-order kinetic model indicates that the mechanism involved in the sorption is governed by ion exchange or the sharing of electrons (Babalola et al., 2019).
In a similar study by Babalola et al. (2019), the calculated Qe value from the pseudo-second-order kinetic model for the adsorption of Pb(II) ions onto Delonix regia pods and leaves is 4.12 and 2.7g mg g−1 respectively. Bansal et al. (2009) reported 2.81 mg g−1 for the sorption of Ni(II) ions onto rice husks.
3.2.3 Initial adsorbate concentration and isotherm experiment
The experiment conducted to study the effect of the initial concentration of adsorbate on the uptake of Ni(II) and Cu(II) ions by Delonix regia pods and leaves showed that the uptake of both metal ions on the two types of adsorbent increased with increasing adsorbate concentration (Fig. 6a). When the adsorbate concentration is increased, there is increased force driving the metal ions to the binding sites of the adsorbents; thus, increased uptake is recorded. Although the uptake per unit gram of adsorbent increased, the opposite (a decrease) was observed with respect to the percentage of metal removed from the solution as the adsorbate concentration increased. This is because the exchangeable sites in the adsorbent structure are saturated as the ratio of the metal ions to the adsorbent increases, resulting in a decrease in the percentage removed (Man et al., 2012). The data obtained in Figs. 5a and 6a also suggested that the pods are better than the leaves and that the adsorption is more favourable onto the pods at all of the concentrations used in this study.
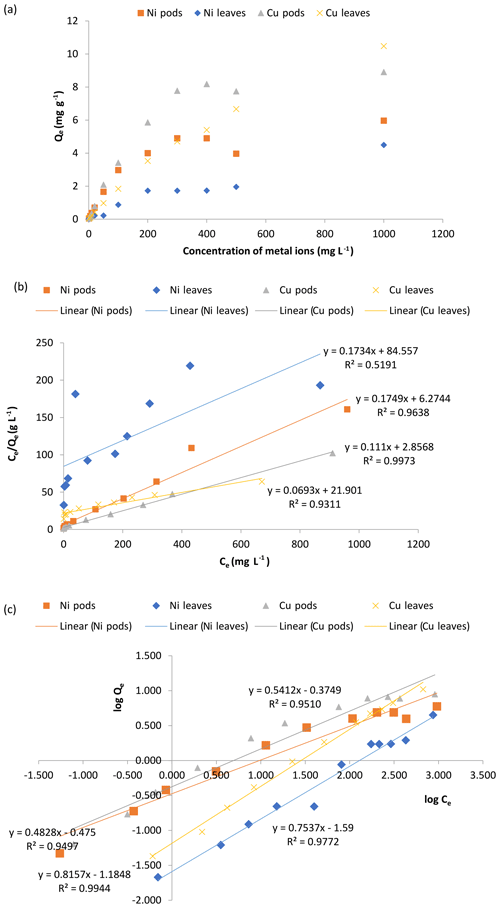
Figure 6The effect of the initial metal ion concentration (a), and plots of the Langmuir (b) and Freundlich (c) isotherms for the adsorption of Ni(II) and Cu(II) ions by Delonix regia pods and leaves. The experimental conditions were as follows: a pH of 4 and 5, a contact time of 30 min, an adsorbent dosage of 0.5 g and a metal ion concentration of 1–1000 mg L−1.
The relatively high concentration of metal ions compared with the adsorbents' available binding surfaces as the concentration is increased could be responsible for the observed decrease in the percentage removal (Meena et al., 2005b; Hamza et al., 2013). The data obtained from this experiment were modelled using the Langmuir and Freundlich isotherm models.
The results presented in Fig. 6b for the Langmuir isotherm model showed that the Langmuir isotherm is favourable for its adsorption of Ni(II) and Cu(II) ions for the pods. The values obtained for the adsorption of the metal ions onto the leaves showed that the adsorption of Cu(II) ions onto the leaves is more favourable than that of Ni(II) ions. The fit to the plot of the Langmuir isotherm suggests possible monolayer coverage of the metal ions on the adsorbent surface except for the sorption of Ni(II) ions to the leaves, where fluctuations were observed. The high correlation coefficient (R2) obtained for the isotherm when sorption was carried out using the Delonix regia pods is also an indication of its applicability in the sorption reaction. The correlation coefficient obtained using Delonix regia leaves as the adsorbent was not as high as that obtained for the pods, implying that the Langmuir isotherm is not the best model to explain the sorption of Ni(II) and Cu(II) onto the leaves. The parameters obtained for the Langmuir isotherm modelling are shown in Table 2.
Table 2The isotherm models' adsorption parameters for the adsorption of Ni(II) and Cu(II) onto Delonix regia.
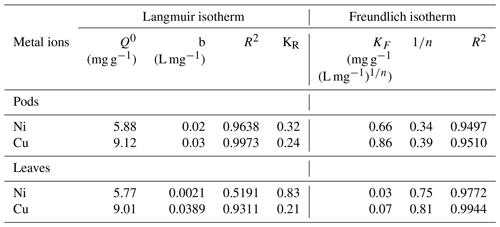
The plot of the Freundlich isotherm is presented in Fig. 6c. Using the Freundlich isotherm model on the data obtained from the concentration study, it was further discovered (from the values of the adsorption parameters shown in Table 2) that the adsorption of Ni(II) and Cu(II) ions onto Delonix regia leaves is more favourable than the adsorption of these metal ions onto the pods. The values obtained for 1∕n were 0.75 and 0.81 for the adsorption of Ni(II) and Cu(II) ions respectively for the leaves, whereas the values for their respective sorption onto the pods were 0.34 and 0.39 respectively. The lower Freundlich constant, 1∕n, values for the pods and the higher adsorption capacity, KF, values are further confirmation that the pods adsorb more effectively than the leaves.
The values obtained for the Freundlich isotherm correlation coefficient (R2) during the adsorption of Ni(II) and Cu(II) ions onto the leaves are higher than those obtained for the adsorption onto the pods. Thus, the Freundlich isotherm is a better isotherm to describe the adsorption of Ni(II) and Cu(II) ions onto Delonix regia leaves, whereas the Langmuir isotherm is better fitted to describe adsorption onto the pods.
Kumar et al. (2019) reported that the sorption of Cu(II) ions onto groundnut seed cake, sesame seed cake and coconut cake powders fit perfectly to the Langmuir isotherm with a Q0 value ranging from 3.608 to 3.703 mg g−1. Likewise, Saeed et al. (2005a) reported that the biosorption of Cu(II) ions perfectly fit the Langmuir adsorption isotherm model. Olufemi and Eniolaodunmo (2018) reported a Q0 of 1.47 and 2.57 mg g−1 for the sorption of Ni(II) ions onto banana peels and coconut shells respectively with a Langmuir constant b (L mg−1) of 0.018 and 0.016 respectively. Malkoc and Nuhoglu (2005) recorded a Q0 of 15.26 mg g−1 and a b value of 0.088 L mg−1 for the adsorption of Ni(II) ions onto tea factory waste. Thus, the isotherm experiment has shown that the Langmuir constant values related to the sorption energy obtained in this study were very close to banana peels, coconut shells and tea waste, except for the adsorption of Ni(II) ions onto the leaves which was lower. Moreover, the Q0 values of the pods and leaves of Delonix regia in this work were higher than those for banana peels and coconut shells but lower than that for tea factory waste.
In a similar study by Babalola et al. (2019), the Langmuir isotherm provided the best fit for the adsorption of Pb(II) ions onto Delonix regia pods, whereas the Freundlich isotherm gave a better fit for the leaves. This implies that the sorption of these metal ions onto the pods assumes a monolayer adsorption onto a homogeneous surface with a finite number of identical sites, whereas sorption onto the leaves assumes that the metal ions adsorb onto the heterogeneous surface of the adsorbent (Ayanda et al., 2013).
3.2.4 Adsorbent dosage and solutions' ionic strength
Figure 7a revealed the results of changing the adsorbent doses on the adsorption of Ni(II) and Cu(II) ions by Delonix regia biomass. It was observed that the percentage adsorption of Ni(II) ions increased from 45.9 % to 80.4 % and from 21.6 % to 33.7 % onto the pods and leaves respectively, whereas the percentage adsorption of Cu(II) ions increased from 72.6 % to 89.2 % and from 69.6 % to 80.8 % onto the pods and leaves respectively. The observed increase in the percentage of Ni(II) and Cu(II) ions adsorbed might be due to the availability of more binding sites as adsorbent doses are increased.
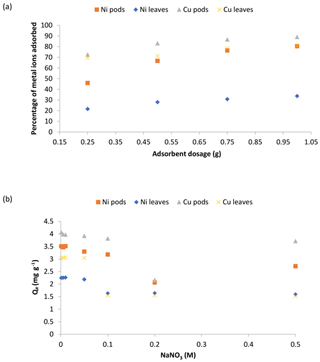
Figure 7The effect of the adsorbent dosage (a) and the ionic strength (b) on the adsorption of Ni(II) and Cu(II) ions by Delonix regia pods and leaves. The experimental conditions were as follows: a pH 4 and 5, a contact time of 30 min and a metal ion concentration of 100 mg L−1.
The results of the effect of the sodium ion concentration on the adsorption of Ni(II) and Cu(II) ions by Delonix regia biomass is shown in Fig. 7b. The figure shows a reduction in the uptake of both metal ions by each of the biomasses. This could be explained by the fact that there was competition for the available binding sites on the adsorbents by the positive sodium ions present in the adsorption medium (Alegbe et al., 2019). Thus, as the concentration of sodium ions is increased, metal uptake by the adsorbent decreases.
A comparison of the adsorption efficiency of different agricultural waste with respect to Ni(II) and Cu(II) ions is presented in Table 3. The results obtained from this study show that Delonix regia biomass competes favourably with other agricultural waste that has been used in previous studies and, hence, could be useful for removing Ni(II) and Cu(II) ions from aqueous solution.
3.3 Desorption study
The regeneration of adsorbents after adsorption is of outmost importance; metal ions adsorbed should be easily desorbed under suitable conditions, and the adsorbents should be used repeatedly to reduce the cost of the material. Thus, a recovery experiment was carried out to investigate the possibility of recovering the adsorbed metal ions from the biomasses. The results obtained from the recovery study are shown in Fig. 8.
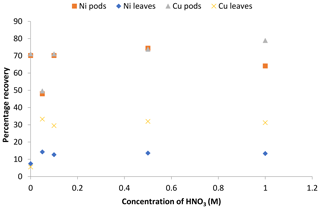
Figure 8Recovery study of the adsorbed Ni(II) and Cu(II) ions from the pods and leaves of Delonix regia.
The values obtained for the percentage recovery of Ni(II) and Cu(II) ions from the Delonix regia pods showed that different percentages of metal ions were recovered at different desorbing medium concentrations (Fig. 8). The results also revealed that a relatively low concentration of nitric acid (0.1 M) could be used to recover more than 50 % of the metal ions from the pods. Moreover, Fig. 8 shows that the percentage of metal ions recovered from the pods was higher than that recovered from the leaves at various nitric acid concentrations. Approximately 74.4 % of Ni(II) ions and 78.9 % of Cu(II) ions were recovered from Delonix regia pods using 1.0 M nitric acid, whereas 14.3 % of Ni(II) ions and 33.3 % of Cu(II) ions were recovered from the Delonix regia leaves with the same nitric acid concentration. The recovery experiment showed that metal ion recovery increased with an increasing concentration of nitric acid. Moreover, Delonix regia biomass, especially the pods, could be reused repeatedly in the removal of heavy metal without losing its adsorption properties.
This study concerns the use of powdered Delonix regia pods and leaves for the removal of Ni(II) and Cu(II) ions from aqueous solutions. The results obtained indicated that the pH of the solution, the contact time, the initial metal ion concentrations, the adsorbent dosage and the ionic strength affect the uptake of the metal ions by the biosorbent. It was observed that the Freundlich isotherm is better fitted to describe the adsorption of Ni(II) and Cu(II) ions onto Delonix regia leaves, whereas the Langmuir isotherm is more suitable to describe the adsorption onto the pods. The pseudo-second-order kinetic modelling results agrees with the sorption of Ni(II) and Cu(II) ions onto Delonix regia pods and leaves. The desorption study also showed that metal ions could be desorbed from spent Delonix regia biomass and that the biomass could be repeatedly reused without losing its adsorption properties. The pods performed better than the leaves in terms of the amount of metal ions removed and the regeneration of the spent adsorbent. In conclusion, the powdered pods and leaves of Delonix regia could be used as eco-friendly, cheap and effective adsorbents for the removal of Ni(II) ions, Cu(II) ions and other environmental contaminants from aqueous solution.
The data generated and/or analysed during the current research are available from the authors upon reasonable request.
BMB was the investigator and contributed to writing the paper. AOB and EOO were involved in the characterization of the adsorbent. COA, OSL and SFA were involved in the adsorption studies. LSA contributed to modelling the data. OSA was involved in modelling the adsorption data and contributed to writing the paper.
The authors declare that they have no conflict of interest.
The authors acknowledge Remy Bucher from the Department of Materials Research at iThemba LABS, South Africa, for the XRD analysis of the pod and leaf samples.
This paper was edited by Luuk Rietveld and reviewed by two anonymous referees.
Abdel-Tawwab, M., El-Sayed, G. O., and Shady, S. H. H.: Capability of some agricultural wastes for removing some heavy metals from polluted water stocked in combination with Nile tilapia, Oreochromis niloticus (L.), Int. Aquat. Res., 9, 153–160, 2017.
Ajmal, M., Rao, R. A. K., Anwar, S., Ahmad, J., and Ahmad, R.: Adsorption studies on rice husk: removal and recovery of Cd (II) from wastewater, Biores. Technol., 86, 147–149, 2003.
Alatabe, M. J.: Adsorption of copper (II) ions from aqueous solution onto activated carbon prepared from cane papyrus, Pollution, 4, 649–662, 2018.
Alegbe, M. J., Ayanda, O. S., Ndungu, P., Nechaev, A., Fatoba, O. O., and Petrik, L. F.: Physicochemical characteristics of acid mine drainage, simultaneous remediation and use as feedstock for value added products, J. Environ. Chem. Eng., 7, 103097, https://doi.org/10.1016/j.jece.2019.103097, 2019.
Anantha, R. K. and Kota, S.: An evaluation of the major factors influencing the removal of copper ions using the egg shell (Dromaius novaehollandiae): chitosan (Agaricus bisporus) composite, 3Biotech., 6, 83, https://doi.org/10.1007/s13205-016-0381-2, 2016.
Ayanda, O. S., Fatoki, O. S., Adekola, F., and Ximba, B. J.: Kinetics and equilibrium models for the sorption of tributyltin to nZnO, activated carbon and nZnO/activated carbon composite in artificial seawater, Mar. Pollut. Bull., 72, 222–230, 2013.
Babalola, B. M., Babalola, A. O., Adubiaro, H. O., Ayanda, O. S., Nelana, S. M., and Naidoo, E. B.: Application of waste Delonix regia pods and leaves for the sorption of Pb(II) ions from aqueous solution: kinetic and equilibrium studies, Water Qual. Res. J. Can., 54, 278–289, 2019.
Bansal, M., Singh, D., Garg, V. K., and Rose, P.: Use of agricultural waste for the removal of Nickel ions from aqueous solutions: equilibrium and kinetics studies, Int. J. Civil Environ. Eng., 1, 108–114, 2009.
Brewer, G. J.: Copper toxicity in the general population, Clin. Neurophysiol., 121, 459–460, 2010.
Brewer, G. J.: Iron and copper toxicity in diseases of aging, particularly atherosclerosis and Alzheimer's disease, Exp. Biol. Med., 232, 323–335, 2007.
Dean, J. G., Bosqui, F. L., and Lanouette, K. H.: Removing heavy metals from waste water, Environ. Sci. Technol., 6, 518–522, 1972.
Faller, P.: Copper and zinc binding to amyloid-β: Coordination, dynamics, aggregation, reactivity and metal-ion transfer, ChemBioChem., 10, 2837–2845, 2009.
Festus, A. A., Elvis, O. A., and Morayo, A. B.: Equilibrium sorption of lead and nickel from solutions by flame of the forest (Delonix regia) pods: kinetics and isothermic study, J. Environ. Prot., 4, 261–269, 2013.
Flemming, C. A. and Trevors, J. T.: Copper toxicity and chemistry in the environment: a review, Water, Air, Soil Pollut., 44, 143–158, 1989.
Garg, U. K., Kaur, M. P., Garg, V. K., and Sud, D.: Removal of Nickel(II) from aqueous solution by adsorption on agricultural waste biomass using a response surface methodological approach, Biores. Technol., 99, 1325–1331, 2008.
Guibal, E., Saucedo, I., Roussy, J., Roulph, C., and Le Cloirec, P.: Uranium sorption by glutamate glucan: A modified chitosan Part II: Kinetic studies, Water SA, 19, 119–119, 1993.
Hamza, I. A., Martincigh, B. S., Ngila, J. C., and Nyamori, V. O.: Adsorption studies of aqueous Pb (II) onto a sugarcane bagasse/multi-walled carbon nanotube composite, Phy. Chem. Earth PT A/B/C, 66, 157–166, 2013.
Hansen, H. K., Arancibia, F., and Gutiérrez, C.: Adsorption of copper onto agriculture waste materials, J. Hazard. Mater., 180, 442–448, 2010.
Hasar, H.: Adsorption of nickel (II) from aqueous solution onto activated carbon prepared from almond husk, J. Hazard. Mater., 97, 49–57, 2003.
Ho, Y. S., Huang, C. T., and Huang, H. W.: Equilibrium sorption isotherm for metal ions on tree fern, Process Biochem., 37, 1421–1430, 2002.
Hureau, C. and Faller, P. A.: β-mediated ROS production by Cu ions: structural insights, mechanisms and relevance to Alzheimer's disease, Biochimie, 91, 1212–1217, 2009.
IARC (International Agency for Research on Cancer): IARC Monograph on the evaluation of carcinogenic risks to humans, Lyans, France, 49, 318–411, 1990.
Inoue, Y. and Munemori, M.: Coprecipitation of mercury (II) with iron (III) hydroxide, Environ. Sci. Technol., 13, 443–445, 1979.
Jeme, E. A.: Controls of manganese, iron, cobalt, nickel, copper and zinc concentrations in soils and water, Adv. Chem. Ser., 7, 33–37, 1968.
Kadirvelu, K., Faur-Brasquet, C., and Cloirec, P. L.: Removal of Cu (II), Pb (II), and Ni (II) by adsorption onto activated carbon cloths, Langmuir, 16, 8404–8409, 2000.
Kadirvelu, K., Kavipriya, M., Karthika, C., Radhika, M., Vennilamani, N., and Pattabhi, S.: Utilization of various agricultural wastes for activated carbon preparation and application for the removal of dyes and metal ions from aqueous solutions, Biores. Technol., 87, 129–132, 2003.
Kadirvelu, K., Thamaraiselvi, K., and Namasivayam, C.: Removal of heavy metals from industrial wastewaters by adsorption onto activated carbon prepared from an agricultural solid waste, Biores. Technol., 76, 63–65, 2001.
Kahraman, S., Dogan, N., and Erdemoglu, S.: Use of various agricultural wastes for the removal of heavy metal ions, Inter. J. Environ. Pollut., 34, 275–284, 2008.
Kovacova, Z., Demcak S., and Balintova, M.: Removal of copper from water solutions by adsorption on spruce sawdust, Proceedings, 16, 52, https://doi.org/10.3390/proceedings2019016052, 2019.
Ksakas, A., Tanji, K., El Bali, B., Taleb, M., and Kherbeche, A.: Removal of Cu (II) ions from aqueous solution by adsorption using natural clays: kinetic and thermodynamic studies, J. Mater. Env. Sci, 9, 1075–1085, 2018.
Kumar, G. V. S. R. P., Malla, K. A., Yerra, B., and Rao, K. S.: Removal of Cu (II) using three low-cost adsorbents and prediction of adsorption using artificial neural networks, Appl. Water Sci., 9, 44, https://doi.org/10.1007/s13201-019-0924-x, 2019.
Larous, S. and Meniai, A. H.: Removal of copper (II) from aqueous solution by agricultural by-products- sawdust, Enrgy. Proced., 18, 915–923, 2012.
Liu, H. and Wang, C.: Chitosan scaffolds for recyclable adsorption of Cu (II) ions, RSC Adv., 4, 3864–3872, 2014.
Malkoc, E. and Nuhoglu, Y.: Investigation of Ni II removal from aqueous solutions using tea factory waste, J. Hazard. Mater., B127, 120–128, 2005.
Man, H. C., Wei, H. C., Zadeh, M. R., and Yusof, M. R. M.: Adsorption potential of unmodified rice husk for boron, Bioresources, 7, 3810–3822, 2012.
Meena, A. K., Kadirvelu, K., Mishraa, G. K., Rajagopal, C., and Nagar, P. N.: Adsorption of Pb (II) and Cd (II) metal ions from aqueous solutions by mustard husk, J. Hazard. Mater., 150, 619–625, 2008.
Meena, A. K., Mishra, G. K., Rai, P. K., Rajagopal, C., and Nagar, P. N.: Removal of heavy metal ions from aqueous solutions using carbon aerogel as an adsorbent, J. Hazard. Mater., 122, 161–170, 2005a.
Meena, A. K., Mishra, G. K., Rajagopal, C., and Nagar, P. N.: Adsorption of Ni (II) and Zn (II) from aqueous solution by various adsorbents, Indian J. Environ. Protect., 25, p. 8, 2005b.
Modi, A., Mishra, V., Bhatt, A., Jain, A., Mansoori, M. H., Gurnany, E., and Kumar, V.: Delonix regia: historic perspectives and modern phytochemical and pharmacological researches, Chin. J. Nat. Medicines, 14, 31–39, 2016.
Montanher, S. F., Oliveira, E. A., and Rollemberg, M. C.: Removal of metal ions from aqueous solutions by sorption onto rice bran, J. Hazard. Mater., 117, 207–211, 2005.
Moodley, K., Singh, R., Musapatika, E. T., Onyango, M. S., and Ochieng, A.: Removal of nickel from wastewater using an agricultural adsorbent, Water SA, 37, 41–46, 2011.
Nath, S. K., Jena, A., and Mishra, S. P.: Removal of cadmium by wood charcoal, Trans. Indian Inst. Metals, 50, 235–239, 1997.
Okoya, A. A., Akinyele, A. B., Ofoezie, I. E., Amuda, O. S., Alayande, O. S., and Makinde, O. W.: Adsorption of heavy metal ions onto chitosan grafted cocoa husk char, Afr. J. Pure Appl. Chem., 8, 147–161, 2014.
Olufemi, B. and Eniolaodunmo, O.: Adsorption of nickel (II) ions from aqueous solution using banana peel and coconut shell. Int. J. Technol., 9, 434–445, 2018.
Onwuka, J. C., Agbaji, E. B., Ajibola, V. O., and Okibe, F. G.: Kinetic studies of surface modification of lignocellulosic Delonix regia pods as sorbent for crude oil spill in water, J. Appl. Res. Technol., 14, 415–424, 2016.
Paulino, A. T., Minasse, F. A., Guilherme, M. R., Reis, A. V., Muniz, E. C., and Nozaki, J.: Novel adsorbent based on silkworm chrysalides for removal of heavy metals from wastewaters, J. Colloid Interface Sci., 301, 479–487, 2006.
Ponnusami, V., Gunasekar, V., and Srivastava, S. N.: Kinetics of methylene blue removal from aqueous solution using gulmohar (Delonix regia) plant leaf powder: multivariate regression analysis, J. Hazard. Mater., 169, 119–127, 2009.
Raji, C., Manju, G. N., and Anirudhan, T. S.: Removal of heavy metal ions from water using sawdust-based activated carbon, India J. Eng. Mater. Sci., 4, 254–260, 1997.
Rao, P. S., Reddy, K. S., Kalyani, S., and Krishnaiah, A.: Comparative sorption of copper and nickel from aqueous solutions by natural neem (Azadirachta indica) sawdust and acid treated sawdust, Wood Sci. Technol., 41, 427–442, 2007.
Saeed, A., Akhter, M. W., and Iqbal, M.: Removal and recovery of heavy metals from aqueous solution using papaya wood as a new biosorbents, Sep. Purif. Technol., 45, 25–31, 2005a.
Saeed, A., Iqbal, M., and Akhtar, M. W.: Removal and recovery of lead (II) from single and multimetal (Cd, Cu, Ni, Zn) solutions by crop milling waste (black gram husk), J. Hazard. Mater., 117, 65–73, 2005b.
Samuel, J., Shanthi, S., and Vashantha, R.: Utilization of agro-waste material as potential adsorbent for removal of Cu(II) and Ni(II) ions from aqueous phase, Indian J. Adv. Chem. Sci., 4, 346–354, 2016.
Sánchez-Polo, M. and Rivera-Utrilla, J.: Adsorbent-adsorbate interactions in the adsorption of Cd (II) and Hg (II) on ozonized activated carbons, Environ. Sci. Technol., 36, 3850–3854, 2002.
Shukla, S. R. and Pai, R. S.: Adsorption of Cu (II), Ni (II) and Zn (II) on modified jute fibres, Biores. Technol., 96, 1430–1438, 2005.
Shukla, S. S., Yu, L. J., Dorris, K., and Shukla, A.: Removal of nickel from aqueous solutions by saw dust, J. Hazard. Mater., B121, 243–246, 2005.
Singh, R., Gautam, N., Mishra, A., and Gupta, R.: Heavy metals and living systems: An overview, Indian J. Pharmacol., 43, p. 246, 2011.
U.S. Department of Health and Human Services (DHHS): Seventh annual report on carcinogens: Summary, Research Triangle Park, DHHS, National Institute of Environmental Health Sciences, NC, USA, 262–269, 1994.
Van Genderen, E. J., Ryan, A. C., Tomasso, J. R., and Klaine, S. J.: Evaluation of acute copper toxicity to larval fathead minnows (Pimephales promelas) in soft surface waters, Environ. Toxicol. Chem., 24, 408–414, 2005.
Witek-Krowiak, A., Szafran, R. G., and Modelski, S.: Biosorption of heavy metals from aqueous solutions onto peanut shell as a low-cost biosorbent, Desalination, 265, 126–134, 2011.
Zhang, X. and Wang, X.: Adsorption and desorption of Nickel(II) Ions from aqueous solution by a lignocellulose/montmorillonite nanocomposite, PLOS One, 10, e0117077. https://doi.org/10.1371/journal.pone.0117077, 2015.
Zhou, Y., Lei, J., Zhang, Y., Zhu, J., Lu, Y., Wu, X., and Fang, H.: Determining discharge characteristics and limits of heavy metals and metalloids for wastewater treatment plants (WWTPs) in China based on statistical methods, Water, 10, 1248, https://doi.org/10.3390/w10091248, 2018.