the Creative Commons Attribution 4.0 License.
the Creative Commons Attribution 4.0 License.
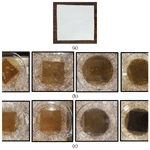
Surface-water purification using cellulose paper impregnated with silver nanoparticles
Shahad A. Raheem
Alaa H. Alfatlawi
The objective of this study is to prepare a cellulose paper that was impregnated with silver nanoparticles (AgNPs) for the purpose of water purification (disinfection and filtration). AgNP papers were prepared by chemical reduction of silver nitrate (AgNO3) with various concentrations (0.005 M, 0.015 M, 0.03 M, and 0.05 M) using sodium borohydride (NaBH4) as a reducing agent. Two ratios for of 2:1 and 10:1 were used to show the effect of reduction on the formation and removal efficiencies of AgNPs. AgNP papers were characterized using scanning electron microscopy and transmission electron microscopy. An acid digestion using HCl acid followed by analyzing the samples in an atomic absorption spectrometer (ASS) was conducted to measure the silver concentration in AgNP papers. TEM images showed that the silver nanoparticle size in the papers varied from 1.3 to 75 nm.
Water samples, after filtration through AgNP papers, were analyzed using ASS to measure the silver concentration in the effluent water. AgNP paper antibacterial efficiency ranged from 99 % to 100 % for both reduction ratios. The average silver content in the effluent water for the three replicates ranged from 0 to 0.082 mg L−1, which meets the United States Environmental Protection Agency (US-EPA) guideline for drinking water of less than 0.1 mg L−1. Turbidity tests showed that these papers can be usefully used as point-of-use filters as the turbidity reduced to less than 1 NTU (Nephelometric Turbidity Units).
Water is the common medium for many pathogens because it contains several bacteria, viruses, etc. The removal and inactivation of pathogenic microorganisms are the last steps in the treatment of drinking water (Phong et al., 2009). Although disinfection methods currently used in drinking water treatment can effectively control microbial pathogens, researches in the past few decades have revealed a dilemma between effective disinfection and formation of harmful disinfection byproducts (DBPs) (Li et al., 2008). When chlorine comes in contact with natural organic matter (NOM), carcinogenic compounds such as trihalomethanes (THMs) and haloacetic acids (HAAs) can be formed (Lalley et al., 2014).
Nanotechnology as well as its application is one of the rapidly developing sciences (Hossain et al., 2014). It is an important field of modern research dealing with the design, synthesis, and manipulation of a particle's structure ranging from approximately 1–100 nm (Korbekandi and Iravani, 2012). Among the most promising nanomaterials with antibacterial properties are metallic nanoparticles, which exhibit increased chemical activity due to their large surface to volume ratios and crystallographic surface structure (Savage and Diallo, 2005). Silver nanoparticles have proved to be most effective as they have good antimicrobial efficacy against bacteria, viruses and other eukaryotic microorganisms (Rai et al., 2009). The investigation of enhanced disinfection through the use of silver nanoparticle (AgNP) surface immobilization has been continually explored. From silver-doped hydroxyapatite coatings for reduced infection rate of implanted biomedical devices (Bai et al., 2012) to silver-impregnated ceramic filters for point-of-use treatment in rural Guatemala (Kallman et al., 2011), AgNP-coated surfaces have displayed a wide range of potential applications.
These commonly encountered materials could be beneficial in maintaining bacteria-free water which is being stored or transported. Graphene, activated carbon and nepheline films have also been studied for AgNP immobilized antibacterial surfaces (Lalley et al., 2014).
Cellulose materials serve as a good material for embedding metal nanoparticles due to their ability for metal ion absorption. Metal cations have an affinity for anionic carboxylic acid groups in paper; the porosity of the base paper allows microorganisms to come into contact with the biocide, but attachment to the fiber surfaces limits the level of silver in the effluent water. The large pore size in the paper allows for reasonably rapid flow by gravity without the need for pressure or suction (Dankovich and Gray, 2011).
The main objective of this study is to prepare disinfection material that has a high antibacterial efficiency, few side effects and can be readily used for water treatment. This study considers the use of nanotechnology, specifically the use of silver nanoparticles (AgNP) for water disinfection purposes (inactivating of Escherichia Coli, Staphylococcus aureus, Enterococcus faecalis, Klebsiella pneumoniae and Enterobacter aerogenes).
2.1 Sampling
The study area was Shatt Al-Hilla river at Al-Hilla city, Iraq, from which the samples were taken during the period 2018 to 2019; this is because this river is the main source of water in the city, and we wanted to investigate the ability of AgNP papers to purify this water. Water sample characteristics are presented in (Table 1). A sample of 500 mL of water was grabbed and kept in precleaned plastic bottles. The samples were analyzed immediately to prevent any change in their quality that may occur.
2.2 Preparation of AgNP papers
A 10 cm × 10 cm × 0.8 mm off-white paper, 100 % alpha cellulose, Whatman Gel Blot GB005, was used to embed silver nanoparticles. AgNP papers were prepared by in situ reduction of AgNO3 with various concentrations (0.005 M, 0.015 M, 0.03 M and 0.05 M) and two reduction ratios of 2:1 and 10:1, to show the effect of increasing the concentration of the reducing agent on the formation of AgNPs. Each paper was soaked in 40 mL of AgNO3 solution for 30 min; then it was washed with ethanol for 1 min to remove the excess Ag ions which were not adsorbed by the paper. To form AgNPs, the paper was placed in 40 mL of NaBH4 solution for 1 h. After that, the paper was soaked in deionized water for 30 min. Then the paper was dried in the oven at 60 ∘C for 2.5 h. Figure 1 shows the papers before and after embedding with AgNPs.
2.3 Characterization
The synthesized AgNP papers were characterized by scanning electron microscopy (SEM), type Quanta 450, available at the University of Babylon (College of Pharmacy) and transmission electron microscopy (TEM) available at Al-Nahrain University (College of Medicine).
2.4 Acid digestion
To determine the amount of dissolved silver in the AgNP paper, an acid digestion of the paper was performed, and then the amount of dissolved silver was analyzed with an atomic absorption spectrometer (AAS, type AA320N) available at the University of Babylon (College of Material Engineering). Approximately 100 mg of the dried AgNP paper was reacted with 5 mL of nitric acid (HNO3) and 5 mL of water. The mixture was boiled until the paper was disintegrated. A volume of 5 mL of 30 % hydrogen peroxide (H2O2) was added to the mixture to assist in the complete oxidation of the organic matter and to release additional metals into the solution. The mixture was boiled again, left to be cooled, and then filtered through Whatman filter paper (grade 41) with a diameter of 15 cm; it was then diluted by adding 100 mL of water. The diluted mixture was tested for silver content using an AAS.
2.5 Microbiological test
Urinary tract infections (UTIs) chromogenic agar was prepared by suspending 47.5 g of the medium in 1 L of distilled water. The mixture was mixed well and dissolved by heating with frequent agitation. Then it was boiled for 1 min until complete dissolution. The medium was sterilized by placing it in an autoclave at 121 ∘C for 15 min; then it was cooled to 45–50 ∘C, mixed well, dispensed into plates and left to solidify. The dehydrated medium was homogeneous, free-flowing and beige in color.
The samples were cultured using the serial dilutions method: 1 mL of the sample was diluted in 9 mL of distilled water (1:10 dilution). This process repeated until a 1:100 000 dilution was reached. A volume of 0.1 mL of each dilution was spread over a medium plate, and then the plates were incubated at 37 ∘C for 48 h in an incubator (LIB-030M). The colonies were counted by eye and colony-forming units per milliliter were calculated using the following equation (Hameed et al., 2015):
3.1 Paper characterization
The AgNP papers were characterized by SEM and TEM. Figure 2 represents the images obtained by SEM to show the presence of AgNPs in paper fibers and the images obtained by TEM to determine the particle sizes of AgNPs. Table 2 represents the particle sizes of AgNPs obtained by TEM.
TEM images and results presented in Table 2 showed that an excess of sodium borohydride reductant (10:1 ratio of sodium borohydride to silver nitrate) resulted in more uniform and smaller nanoparticles. This can be due to the increased speed of reduction with the increment in the reducing agent (Quintero-Quiroz et al., 2019) . These results agree with the findings of the previous studies concerning this subject.
3.2 Acid digestion
Acid digestion was performed to determine the silver content of the paper. The results were obtained by using AAS (type AA320N). Table 3 shows the results of the AAS test.
The acid digestion of AgNP papers showed silver content ranging from 3.9 to 8.7 mg Ag g−1 (milligram Ag per dry gram of paper). The increase in silver content of the paper correlates with the increase in precursor silver ion concentration of the solution in which the papers were soaked, prior to reduction (Dankovich and Gray, 2011). For the same concentration of AgNO3, the ratio of 10:1 resulted in more silver content than the 2:1 ratio. These results agree with the findings of the previous studies concerning this subject.
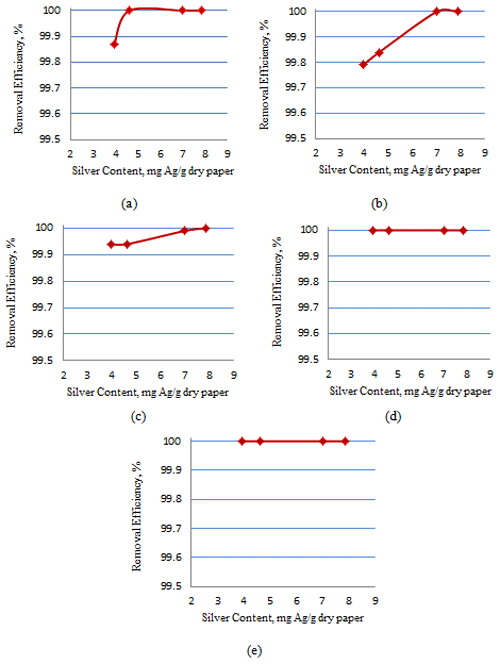
Figure 3The removal efficiency of different types of bacteria with ratio of 2:1: (a) E. coli, (b) Staphylococcus aureus, (c) Enterococcus faecalis, (d) Enterobacter aerogenes and Klebsiella pneumoniae, and (e) Proteus mirabilis.
3.3 Removal efficiencies of bacteria
Figures 3 and 4 show the effect of the silver content in the AgNP paper on the removal efficiency of different types of bacteria with a ratio of 2:1 and 10:1, respectively.
As shown in Figs. 3 and 4, the minimal inhibitory concentration (MIC), which is the lowest concentration of silver nanoparticles needed to inactivate the bacteria, for E. coli for all three filtration times was 4.62 mg Ag g−1 dry paper for a ratio of 2:1, while for 10:1, the MIC for complete inactivation of E. coli was 4.34 mg Ag g−1 dry paper. The MIC for complete inactivation of Staphylococcus aureus was 7.01 mg Ag g−1 dry paper for 2:1 ratio and 4.7 mg Ag g−1 dry paper for 10:1 ratio. The MIC for complete inactivation for Enterococcus faecalis was 4.01 mg Ag g−1 dry paper for 2:1 ratio and 4.7 mg Ag g−1 dry paper for 10:1 ratio. The removal efficiency for Enterobacter aerogenes and Klebsiella for all silver contents and both ratios was 100 %.
It was observed that for all types of bacteria, the ratio of 10:1 resulted in complete inactivation of bacteria in less silver content than the 2:1 ratio and that the 10:1 ratio resulted in smaller and more uniform AgNPs, which led to better contact between the AgNPs and the bacteria (Bakhtiari-Sardari et al., 2020). These results agree with the findings of the previous studies concerning this subject.
3.4 Analysis of silver content in the effluent
Due to possible human health effects from silver exposure, the silver content in the effluent water was analyzed by AAS. Table 4 represents the relationship between the silver content in the paper and silver release in the effluent.
As shown in Table 4, the average silver content in the effluent water for the three replicates ranged from 0 to 0.082 mg L−1, which meets the United States Environmental Protection Agency (US-EPA) guideline for drinking water of less than 0.1 mg L−1 (EPA, 2018). This was due to the stability of silver nanoparticle in the cellulose paper. Sodium borohydride acts not only as a reducing agent but also as an ion stabilizer, which prevents silver ions from aggregation. Moreover, hydroxyl and ether groups in the cellulose fiber play an important role in the stabilization of metal nanoparticles (Pinto et al., 2012). These results agree with the findings of the previous studies concerning this subject.
3.5 Turbidity removal
Turbidity tests were conducted using a turbidity meter, SN 10/1467, Germany, available at the University of Babylon (College of Engineering, Environmental Engineering Department). Table 5 represents the results obtained before and after filtration through AgNP papers.
As shown in Table 5, the cellulose paper acts as a good point-of-use filter as all the turbidities were reduced to an acceptable level. Reducing the papers with the 10:1 ratio reduced the turbidity better than the 2:1 ratio.
Sliver nanoparticles used in this work exhibit a broad size distribution with highly reactive facets. It was observed that chemical reduction of AgNO3 by using NaBH4 as a reducing agent resulted in spherical silver nanoparticles. The ratio for of 10:1 resulted in smaller sizes of silver nanoparticle and more silver content than the ratio of 2:1 for the same AgNO3 concentration. AgNP paper provided rapid and effective bactericidal activity as the bacterially contaminated water was filtered through the paper; this is especially important for Enterococcus faecalis, which is for the first time tested for removal by AgNPs. AgNP papers can be used as good point-of-use filters.
AgNPs | silver nanoparticles |
AgNO3 | silver nitrate |
NaBH4 | sodium borohydride |
SEM | scanning electron microscopy |
TEM | transmission electron microscopy |
AAS | atomic absorption spectrometer |
DBPs | disinfection byproducts |
NOM | natural organic matter |
THMs | trihalomethanes |
HAA | haloacetic acid |
TDS | total dissolved solid |
HCl | hydrochloric acid |
HNO3 | nitric acid |
H2O2 | hydrogen peroxide |
UTI | urinary tract infections |
MIC | minimal inhibitory concentration |
No data sets were used in this article.
SAR has done samples and data collection and lab experiments as well as writing and editing the paper. AHA role was supervising the process of data and samples collection and experiments and made the final correction to writing.
The authors declare that they have no conflict of interest.
This study was supported by the Department of Environmental Engineering in the University of Babylon. We also appreciate the support of the sanitary lab in the College of Engineering that helped carry out this work.
This paper was edited by Luuk Rietveld and reviewed by Mona Soliman and one anonymous referee.
Bai, X., Sandukas, S., Appleford, M., Ong, J. L., and Rabiei, A.: Antibacterial effect and cytotoxicity of Ag-dopedfunctionally graded hydroxyapatite coatings, J. Biomed. Mater. Res. B, 100B, 553–561, 2012.
Bakhtiari-Sardari, A., Mashreghi, M., Eshghi, H., Behnam-Rasouli, F., Lashani, E., and Shahnavaz, B.: Comparative evaluation of silver nanoparticles biosynthesis by two cold-tolerant Streptomyces strains and their biological activities, Biotechnol. Lett., https://doi.org/10.1007/s10529-020-02921-1, in press, 2020.
Dankovich, T. A. and Gray, D. G.: Bactericidal Paper Impregnated with Silver Nanoparticles for Point-of-Use Water Treatment, Environ. Sci. Technol., 45, 1992–1998, https://doi.org/10.1021/es103302t, 2011.
EPA: EPA 822-F-18-001, in: 2018 Edition of the Drinking Water Standards and Health Advisories, Office of Water, US Environmental Protection Agency Washington, DC, 2018.
Hameed, U., Muhammad, A., Jahngeer, A.; and ul Haq, I.: Determination of Microbial load of Drinking Water from different areas of Lahore, Biologia (Pakistan), 61, 151–156, 2015.
Hossain, F., Perales-Perez, O. J., Hwang, S., and Román, F.: Antimicrobial nanomaterials as water disinfectant: applications, limitations and future perspectives, Sci. Total Environ., 466–467, 1047–1059, 2014.
Kallman, E. N., Oyanedel-Craver, V. A., and Smith, J. A.: Ceramic filters impregnated with silver nanoparticles for point-of-use water treatment in rural Guatemala, J. Environ. Eng., 137, 407–415, 2011.
Korbekandi, H. and Iravani, S.: Silver Nanoparticles, InTech, Intechopen, 978-953-51-0615-9, https://doi.org/10.5772/34157, 2012.
Lalley, J., Dionysios, D., Varma, R. S., Shankara, S., Yang, D. J., and Nadagouda, M. N.: Silver-based antibacterial surfaces for drinking water disinfection – an overview, Curr. Opin. Chem. Eng., 3, 25–29, 2014.
Li, Q., Mahendra, S., Lyon, D. Y., Brunet, L., Liga, M. V., Li, D., and Alvarez, P. J.: Antimicrobial nanomaterials for water disinfection and microbial control: potential applications and implications, Water Res., 42, 4591–4602, https://doi.org/10.1016/j.watres.2008.08.015, 2008.
Phong, N. T., Thanh, N. K., and Phuong, P. H.: Fabrication of antibacterial water filter by coating silver nanoparticles on flexible polyurethane foams, J. Phys. Conf. Ser., 187, 012079, https://doi.org/10.1088/1742-6596/187/1/012079, 2009.
Pinto, R. J. B., Neves, M. C., Pascoal Neto, C., and Trindade, T.: Composites of Cellulose and Metal Nanoparticles, in: Nanocomposites – New Trends and Developments, edited by: Ebrahimi, F., IntechOpen, https://doi.org/10.5772/50553, 2012.
Quintero-Quiroz, C., Acevedo, N., Zapata-Giraldo, J., Botero, L. E., Quintero, J., Zárate-Triviño, D., Saldarriaga, J., and Pérez, V. Z.: Optimization of silver nanoparticle synthesis by chemical reduction and evaluation of its antimicrobial and toxic activity, Biomater. Res., 24, 53–67, https://doi.org/10.1186/s40824-019-0173-y, 2019.
Rai, M., Yadav, A., and Gade, A.: Silver nanoparticles as a new generation of antimicrobials, Biotechnol. Adv., 27, 76–83, 2009.
Savage, N. and Diallo, M. S.: Nanomaterials and water purification: Opportunities and challenges, J. Nanopart. Res., 7, 331–342, 2005.