the Creative Commons Attribution 4.0 License.
the Creative Commons Attribution 4.0 License.
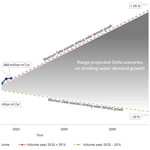
Sustainability characteristics of drinking water supply in the Netherlands
Jolijn Engelenburg
Erik Slobbe
Adriaan J. Teuling
Remko Uijlenhoet
Petra Hellegers
Developments such as climate change and a growing demand for drinking water threaten the sustainability of drinking water supply worldwide. To deal with this threat, adaptation of drinking water supply systems is imperative, not only on a global and national scale but particularly on a local scale. This investigation sought to establish characteristics that describe the sustainability of local drinking water supply. The hypothesis of this research was that sustainability characteristics depend on the context that is analysed, and therefore, a variety of cases must be analysed to reach a better understanding of the sustainability of drinking water supply in the Netherlands. Therefore, three divergent cases on drinking water supply in the Netherlands were analysed. One case related to a short-term development (2018 summer drought), and two concerned long-term phenomena (changes in water quality and growth in drinking water demand). We used an integrated systems approach, describing the local drinking water supply system in terms of hydrological, technical, and socio-economic characteristics that determine the sustainability of a local drinking water supply system. To gain a perspective on the case study findings that are broader than the Dutch context, the sustainability aspects identified were paired with global aspects concerning sustainable drinking water supply. This resulted in the following set of hydrological, technical, and socio-economic sustainability characteristics: (1) water quality, water resource availability, and impact of drinking water abstraction; (2) reliability and resilience of the technical system and energy use and environmental impact; (3) drinking water availability, water governance, and land and water use. Elaboration of these sustainability characteristics and criteria into a sustainability assessment can provide information on the challenges and trade-offs inherent in the sustainable development and management of a local drinking water supply system.
Climate change, combined with a growing drinking water demand, threatens the sustainability of the drinking water supply worldwide. The goal set for drinking water supply in Sustainable Development Goal (SDG) 6.1 (UN, 2015) is “to achieve universal and equitable access to safe and affordable drinking water for all by 2030”. Worldwide drinking water supply crises are visible, resulting from a combination of limited water resource availability, lacking or failing drinking water infrastructure, and/or increased drinking water demand due to short-term events or long-term developments (WHO, 2017). Still, nearly 10 percent of the world population is fully deprived of improved drinking water resources (Ekins et al., 2019), and additionally, existing drinking water supply systems are often under pressure. For instance, two recent examples of water crises were reported in Cape Town, South Africa, and São Paolo, Brazil (Sorensen, 2017; Cohen, 2016). To deal with such challenges and threats to safe and affordable drinking water, adaptation of the current drinking water supply system is imperative, not only on a global and national level but also on a local scale.
In the Netherlands, for instance, the national drinking water supply currently meets the indicator from SDG 6 (UN, 2018) on safely managed drinking water services and safely treated wastewater. At the same time, the more specific goals on (local) water quantity, quality, and ecology, as set by the European Water Framework Directive (WFD), are not met yet (European Environment Agency, 2018). Consequently, drinking water supply in the Netherlands does not meet all SDG 6 indicators, for instance when considering impact on water-related ecosystems (Van Engelenburg et al., 2018), of water pollution (Kools et al., 2019; Van den Brink and Wuijts, 2016), or of water shortage (Ministry of Infrastructure and Environment and Ministry of Economic Affairs and Climate Policy, 2019). Additionally, future developments, such as the uncertain drinking water demand growth rate (Van der Aa et al., 2015) and the changing climate variability (Teuling, 2018), may put the sustainability of the Dutch drinking water supply under pressure in the future.
The abstraction of groundwater or surface water from the hydrological system, and subsequent treatment to drinking water quality before being distributed to customers, requires local infrastructure (typically a drinking water production facility embedded in a distribution network of pipelines). Although the daily routine of drinking water supply has a highly technical character (Bauer and Herder, 2009), the sustainability in the long-term depends on the balance between technical, socio-economic, and environmental factors. This balance is especially complex for the local drinking water supply, which is intertwined with the local hydrological system and local stakeholders through its geographical location.
Because of the interconnections between physical, technical, and socio-economic factors as well as across space, organizational levels, and time, adaptation of the local drinking water supply to current and future sustainability challenges calls for an integrated planning approach (Liu et al., 2015). Integrated models have been developed to understand the complex interactions between the physical, technical, and socio-economic components in various water systems (Loucks et al., 2017). However, a systems analysis to assess local drinking water supply and to identify sustainability challenges on a local scale has not yet been developed.
This research aimed to propose a set of sustainability characteristics that describe the drinking water supply system on a local scale to support policy- and decision-making on sustainable drinking water supply. To reach this aim, cases on drinking water supply were analysed using a conceptual framework. The selected cases represented a short-term event and long-term developments that affect water quality and water resource availability, the technical drinking water supply infrastructure, and/or the drinking water demand. The system boundaries were set to drinking water supply on a local scale. While the drinking water supply on a local scale is also affected by outside influences from different organizational and spatial scales, the analysis accounted for these external influences too. The hypothesis of this research was that sustainability characteristics depend on the context that is analysed, and therefore, a variety of cases must be analysed to reach a better understanding of the sustainability of drinking water supply in the Netherlands.
Sustainable water systems can be defined as water systems that are designed and managed to contribute to the current and future objectives of society, maintaining their ecological, environmental, and hydrological integrity (Loucks, 2000). This study focused on the sustainability of drinking water supply systems on a local scale – in short, local drinking water supply systems. The boundaries of these systems were set by the area in which drinking water abstraction is embedded. The system can be approached from different perspectives. The socio-ecological approach considers relations between the socio-economic and environmental system, whereas the socio-technical approach considers the socio-economic and technical system (Pant et al., 2015). In this study, we combined both approaches by describing the local drinking water supply system in terms of hydrological, technical, and socio-economic characteristics that determine the sustainability of a local drinking water supply system.
Drinking water supply in the Netherlands is of a high standard compared to many other countries. The SDG 6 targets on safe and affordable drinking water and sanitation and wastewater treatment are basically met. But the Dutch government and drinking water suppliers are also challenged to meet the other goals set in SDG 6, such as the improvement of water quality, increase in water-use efficiency, and protection and restoration of water-related ecosystems. In addition the standards on water quantity, quality, and ecology, as set by the European Water Framework Directive (WFD), have not been achieved yet (European Environment Agency, 2018).
The adopted research approach consisted of four steps. The first step was the selection and analysis of three drinking water practice cases in the Netherlands, aiming to identify the main Dutch sustainability aspects in these cases. Three Dutch cases were selected based on their impact on the sustainability of drinking water supply in the Netherlands, considering a short-term event with limited water resource availability and long-term, ongoing developments on water quality, and growing drinking water demand and water resource availability. The cases are illustrated with Vitens data (Van Engelenburg et al., unpublished, 2020).
In the second step, the cases were analysed using the DPSIR framework (Driver, Pressure, State, Impact, Response; Eurostat, 1999; see Sect. 2.1). The sustainability aspects of these cases were identified in the descriptive results of the DPSIR analysis. The results were combined with Dutch governmental reports on these events and developments (Ministry of Infrastructure and Environment and Ministry of Economic Affairs and Climate Policy, 2019; Vitens, 2016) and cross-checked with Vitens staff. The sustainability aspects were categorized into hydrological, technical, and socio-economic aspects. This resulted in a set of relevant sustainability aspects, which is presented in Appendices A–C. The following step was used to broaden the perspective from the drinking water supply in the Netherlands to a more general perspective by cross-checking the set of sustainability aspects with the targets and indicators in Sustainable Development Goal 6 (hereafter referred to as SDG 6; see Appendix D; UN, 2015) and the WHO Guidelines for Drinking-water Quality (WHO, 2017). The sustainability aspects, as identified in the analysis, were categorized into nine hydrological, technical, and socio-economic sustainability characteristics. In the final step of the study, each sustainability characteristic was elaborated further into five sustainability criteria that describe the local drinking water supply system. The results are described in Sect. 3. A detailed description of the resulting sustainability criteria is presented in Appendix E.
2.1 Case analysis method
To reach the aim of this research to support policy development on sustainable drinking water supply, three practice cases were analysed to identify the main sustainability aspects in these cases using the DPSIR (Driver, Pressure, State, Impact, Response) systems approach (Eurostat, 1999). Drivers describe future developments, such as climate change and population growth. Pressures are developments (in emissions or environmental resources) as a result of the drivers. The state describes the system state that results from the pressures. In this research, the aim is to describe the system state of the drinking water supply system in terms of local hydrological, technical, and socio-economic sustainability characteristics (see Sect. 2.1). The changes in system state cause impacts on system functions, which will lead to societal responses. DPSIR was originally developed to describe causal relations between human actions and the environment. It has also frequently been used for relations and interactions between technical infrastructure and the socio-economic and physical domain (Pahl-Wostl, 2015; Hellegers and Leflaive, 2015; Binder et al., 2013).
The DPSIR approach was used for the analysis of the three selected drinking water supply cases to obtain an overview of the impact of drivers, pressures, and responses to the state of the drinking water supply system. Although the framework has been applied on different spatial scales, Carr et al. (2009) recommend using the framework in a place-specific manner to ensure that local stakeholder perspectives are assessed as well. With the research focus at the local drinking water supply system, these local perspectives were implicitly included. The drivers, pressures, and responses can be on local and higher organizational and/or spatial scales, thus ensuring that – where essential – relevant higher scales are accounted for too.
DPSIR has previously been used for complex water systems by various well-known researchers in this field, such as Claudia Pahl-Wostl. In Binder et al. (2013), a comparison was made between various frameworks, which concluded that DPSIR is a policy framework that does not explicitly include development of a model but aims at providing policy-relevant information on pressures and responses on different scales. In Carr et al. (2009), the use of DPSIR for sustainable development was evaluated. Although the authors were critical regarding the use of the DPSIR framework on national, regional, or global scales, they considered application on a local scale appropriate. They concluded that practitioners could use DPSIR for local-scale studies because it assesses the place-specific nuances of multiple concerned stakeholders more realistically. In Van Noordwijk et al. (2020), DPSIR was used to understand the joint multiscale phenomena in the forest–water–people nexus and, thus, diagnosed issues to be addressed in local decision-making. Therefore, DPSIR was considered an appropriate framework for meeting the aim of the research.
The impact of developments on different temporal scales to the drinking water supply system must be considered as well. The long-lived, interdependent drinking water supply infrastructure is resistant to change due to design decisions in the past which cause path dependencies and lock-ins (Melese et al., 2015). In addition, consumer behaviour, governance and engineering, and the interaction between these processes cause lock-in situations that limit the ability to change towards more sustainable water resources management (Pahl-Wostl, 2002). For this reason, the case analysis was performed considering both short- and long-term pressures, impacts and responses.
2.2 Case selection
In this research, three drinking water supply cases in the Netherlands were selected. The case studies were analysed to find sustainability aspects caused by the identified pressures and short- and/or long-term responses in each case because short-term shocks have different impacts and call for other responses than long-term stresses (Smith and Stirling, 2010). The cases therefore focused on short-term events and long-term developments. All three cases also related to targets set in SDG 6 (UN, 2015). The DPSIR analysis of the case studies is presented in Appendices A–C.
2.3 Case 1: 2018 summer drought
Summer 2018 in the Netherlands was extremely warm and dry, causing water shortages in the water system and a long period of extreme daily drinking water demand, resulting in a record monthly water demand in July 2018 (Ministry of Infrastructure and Environment and Ministry of Economic Affairs and Climate Policy, 2019; see Illustration case 1). The driver in this case is the extreme weather condition, which caused several pressures, such as high temperatures, high evaporation, and a lack of precipitation. These pressures did not only cause drought damage to nature, agriculture, and gardens and parks as well as limited water availability in the surface water and groundwater systems, they also resulted in an extremely high drinking water demand. Data on drinking water supply volumes (Van Engelenburg et al., unpublished, 2020) showed that the extreme drinking water demand during summer 2018 put the drinking water supply system under high pressure, causing extreme daily and monthly drinking water supply volumes that exceeded all previously supplied volumes (see Fig. 1). The capacity of the system was fully exploited but faced limitations in abstraction, treatment and distribution capacity.
Illustration case 1: 2018 summer drought
Within the Vitens supply area, the average daily supply volume during the summer period June–August over the years 2012–2017 was approximately 965 000 m3 d−1. During the period 27 June–4 August 2018, the daily supply volume exceeded this average summer volume by approximately 28 %, with an average volume of nearly 1 240 000 m3 d−1 (Fig. 1a). On 25 July 2019, the maximum daily water supply reached nearly 1 390 000 m3 d−1, which was 42 % above the baseline daily supply (Fig. 1a). The monthly drinking water supply volume in July 2018 of 38 million m3 per month was an increase of 18 % compared to the previous maximum monthly supply volumes (Fig. 1b). Although the drinking water supply infrastructure was designed with an overcapacity to meet the regular demand peaks, the flexibility to more extreme peaks or to long periods of peak demand is limited.
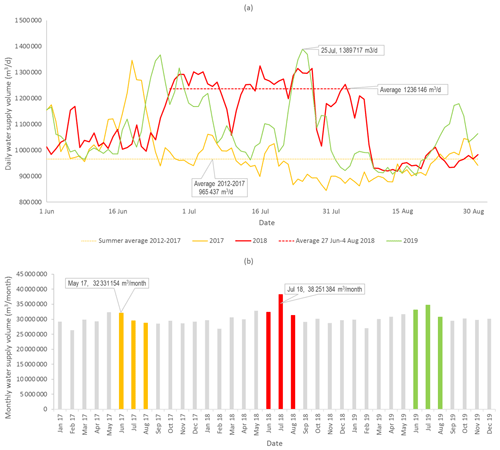
Figure 1Daily (a) and monthly (b) drinking water supply volume by Dutch drinking water supplier Vitens during summer 2017 (average), 2018 (extreme), and 2019 (high) (Van Engelenburg et al., unpublished, 2020).
The high drinking water abstraction volumes added up to the water shortages in both the groundwater and the surface water system that was caused by the lack of precipitation and high evaporation during the summer (Ministry of Infrastructure and Environment and Ministry of Economic Affairs and Climate Policy, 2019). To ensure an acceptable surface water quality for the drinking water supply, measures were taken to reduce salinization (Ministry of Infrastructure and Environment and Ministry of Economic Affairs and Climate Policy, 2019).
To reduce the drinking water use, a call for drinking water saving was made, and locally, pressures in the drinking water distribution system were intentionally lowered to reduce the delivered drinking water volumes (Ministry of Infrastructure and Environment and Ministry of Economic Affairs and Climate Policy, 2019). The problems caused by the summer drought raised a discourse on (drinking) water use and saving, including discussions on controversial measures such as a progressive drinking water tariffs, with tariffs dependent on the consumed drinking water volume and differentiation between high-grade and low-grade use of (drinking) water (Ministry of Infrastructure and Environment and Ministry of Economic Affairs and Climate Policy, 2019). The results of this case analysis are presented in Appendix A.
2.4 Case 2: groundwater quality development
This case focused on the impact of the groundwater quality development in the Netherlands on the drinking water supply. Analysis of the state of the resources for drinking water supply in the Netherlands in 2014 pointed out that, although the drinking water quality met the Dutch legal standards, all water resources are under threat by known and new pollutants (Kools et al., 2019). In the Netherlands, 55 % of the drinking water supply is provided by groundwater resources (Baggelaar and Geudens, 2017). Long-term analysis of water quality records of Dutch drinking water supply fields shows that the vulnerability of groundwater resources to external influences, such as land use, strongly depends on hydrochemical characteristics (Mendizabal et al., 2012). Monitoring results show that, currently, groundwater quality is mainly under pressure due to nitrate, pesticides, historical contamination, and salinization (Kools et al., 2019). Nearly half of the groundwater abstractions for drinking water are affected by an insufficient groundwater quality, and it is expected that, in the future, the groundwater quality at more abstractions will exceed the groundwater standards set in the European Water Framework Directive (European Union, 2000). In addition, traces of pollutants such as recent industrial contaminants, medicine residues, and other emerging substances have been found, indicating that the groundwater quality will likely further deteriorate (Kools et al., 2019).
Groundwater protection regulations regarding land and water use by legal authorities will help to slow down groundwater deterioration (Van den Brink and Wuijts, 2016). However, strategies to restore groundwater quality will often not be effective in the short term because already existing contaminations may remain present for a long period of time, depending on the local hydrological characteristics (Jørgensen and Stockmarr, 2009; see Illustration case 2). The impact of contamination cannot be undone unless soil processes help to (partially) break down contaminants. Thorough monitoring for pollution is therefore essential for following groundwater quality trends and for responding adequately to these trends (Janža, 2015). Due to the expected deterioration of the raw water quality1, different and more complex treatment methods are necessary to continuously meet the drinking water standards (Kools et al., 2019). In general, a more complex treatment method leads to higher energy use, use of additional excipients, water loss, and the production of waste materials, which will lead to a higher water tariff and to a higher environmental impact (Napoli and Garcia-Tellez, 2016). The results of the analysis are presented in Appendix B.
Illustration case 2: groundwater quality development
In the 1980s, the Dutch government implemented regulations to protect water quality by limiting the growing nitrate and phosphate surplus due to overuse of livestock manure. This resulted in a decrease in the nitrate surplus from 1985 on. However, due to the long travel times in groundwater, it took years before the impact of these regulations became visible in the groundwater quality. Figure 2 illustrates the period of time in which the nitrate concentration in an abstraction well still increased despite the 1985 regulations on reduction in the nitrate surplus at surface level. The nitrate concentration in this well increased until 2005 before the nitrate level started to decrease. Only since 2014 has the concentration dropped below the nitrate standard for groundwater of 50 mg L−1.
2.5 Case 3: drinking water demand growth
Due to drinking-water-saving strategies, the drinking water use in the Netherlands per person has decreased from 137 litre per person per day in 1992 to 119 litre per person per day in 2016 (Van Thiel, 2017). This development resulted in a decreasing total yearly drinking water demand volume in that same period, despite the population growth in the Netherlands (Baggelaar and Geudens, 2017). However, 2013 was a turning point at which the total yearly drinking water demand volume in the Netherlands started to grow again (Baggelaar and Geudens, 2017). The trend in the period 2013–2019 shows a strong increase in drinking water demand (see Illustration case 3). Delta scenarios have been developed for the Netherlands, projecting a drinking water demand development varying between a decrease of 10 % to an increase of 35 % in 2050 compared to 2015 (Wolters et al., 2018).
The drinking water demand growth rate for the period 2013–2019, as is seen within the Vitens supply area, compares to the growth rate in the maximum delta scenario of 35 % growth from 2015 to 2050 (See Illustration case 3).
Illustration case 3: drinking water demand growth
The increase in normalized drinking water supply volume as supplied by Vitens between 2015 and 2019 is 4.5 % (Fig. 3). Due to this recent demand growth, the reserve capacity within the existing drinking water supply infrastructure is already limited. The drinking water demand growth rate for the period 2015–2019 compares to the growth rate in the maximum Delta scenario of 35 % growth from 2015 to 2050 (Fig. 3). If this growth rate is not tempered through a significant reduction in the drinking water use, this would require a large extension of the drinking water supply infrastructure.
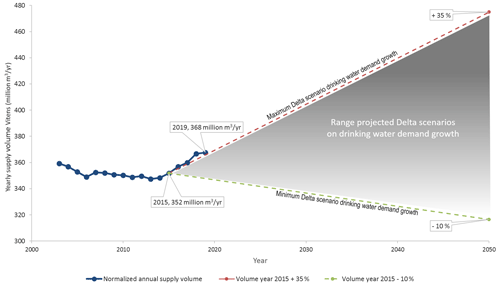
Figure 3Development of the normalized annual drinking water volume supplied by Vitens (drinking water supplier), the Netherlands, 2003–2019 (Van Engelenburg et al., unpublished, 2020), compared to the projected Delta scenarios on drinking water demand growth (Wolters et al., 2018), ranging between a decrease of 10 % and an increase of 35 % in 2050 compared to 2015. The normalized annual drinking water supply volume excludes the impact of extreme weather conditions on the actual supplied annual volumes of drinking water.
If this strong growth rate continues, it will put serious pressure on the drinking water supply. This will partially be due to limitations in the technical infrastructure but also partially due to limitations in the water resource availability caused by insufficient abstraction permits or a possibly negative impact on the hydrological system and stakeholders. Given the inflexibility of drinking water supply infrastructure to change, an integrated strategy is necessary to meet this uncertain development in the drinking water demand. To find sustainable solutions for the future, not only the technical infrastructure aspects must be solved. It also requires strategies on water saving, expansion of permits, development of new abstraction concepts using other water resources, as well as stakeholder processes in the design and use of the local drinking water supply system. This case is basically an extension of the first two cases in that the growing water demand amplifies the aspects caused by the drought in 2018 and the groundwater quality development. The results of the analysis of this case study are presented in Appendix C.
In this section, the sustainability characteristics are presented, each elaborated further into five sustainability criteria. A detailed description of the resulting sustainability criteria can be found in Appendix E.
3.1 Hydrological sustainability characteristics
The following three hydrological sustainability characteristics are proposed that summarize the hydrological aspects affecting the drinking water supply as found in the case studies: water quality, water resource availability, and impact of drinking water abstraction (Table 1).
Table 1Summary of proposed hydrological sustainability characteristics, hydrological aspects from case studies (see Appendices A–C), relevant SDG∗ indicators and WHO Guidelines for Drinking-water Quality (WHO, 2017) aspects, and hydrological sustainability criteria.
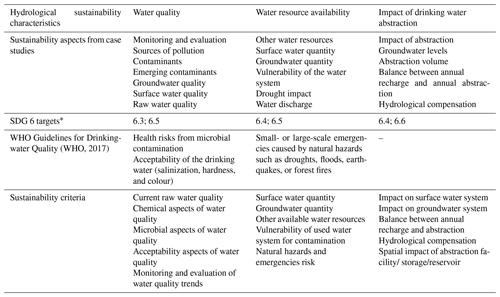
∗ SDG –Sustainable Development Goal; see Appendix V for a summary of SDG 6 targets and indicators related to sustainability characteristics (UN, 2015).
Water quality includes the monitoring and evaluation of current water quality and the trends and expected future development of the water quality and emerging contaminants, as described in the case of “groundwater quality development”. In the WHO Guidelines for Drinking-water Quality (WHO, 2017), the importance of microbial aspects as a global water quality aspect with a health impact is additionally monitored, such as bacteriological contamination due to untreated wastewater or emergencies. The WHO Guidelines for Drinking-water Quality (WHO, 2017) also require monitoring of water quality aspects without a health impact, such as salinization, water hardness, and colour, which affect the acceptability of the drinking water (WHO and UNICEF, 2017).
Water resource availability for drinking water supply can be differentiated into the surface water and groundwater availability, as illustrated in case 1 – “2018 Summer drought”. Other sustainability aspects are the vulnerability of the surface and/or groundwater system to the water quality being permanently affected by land use, as illustrated in the case of “groundwater quality development”. The water resource availability can also be limited due to small- or large-scale emergencies caused by natural hazards, such as droughts, floods, earthquakes, or forest fires (WHO and UNICEF, 2017) that will put the sustainability of the local drinking water supply under pressure.
The impact of the drinking water abstraction on the hydrological system entails the impact on both the surface water system and the groundwater system and also the balance between the annual drinking water abstraction volume and the annual recharge of the (local) water system. Whether the impact of the abstraction is or can possibly be hydrologically compensated is another sustainability aspect. The spatial impact of the local drinking water abstraction facility may also be a sustainability aspect because a drinking water facility requires a certain water storage area or reservoir, which might have a significant spatial impact in the area and, thus, might affect local stakeholders.
3.2 Technical sustainability characteristics
The following three technical sustainability characteristics are proposed that summarize the technical aspects for the drinking water supply as found in the case studies: reliability and resilience of the technical infrastructure and energy use and environmental impact of the drinking water supply (Table 2).
Table 2Summary of proposed technical sustainability characteristics, technical aspects from case studies (see Appendices A–C), relevant SDG∗ indicators and WHO Guidelines for Drinking-water Quality (WHO, 2017) aspects, and technical sustainability criteria.
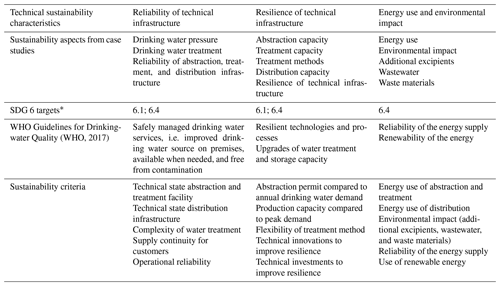
∗ SDG – Sustainable Development Goal; see Appendix V for a summary of SDG 6 targets and indicators related to sustainability characteristics (UN, 2015).
The reliability of the supply system is defined in this research as “the (un)likeliness of the technical system to fail” (Hashimoto et al., 1982). The current technical state of the drinking water production facility and the distribution infrastructure and the complexity of the water treatment are important technical sustainability criteria for the local drinking water supply system. Other technical criteria that should be considered are the supply continuity of the facility, which stands for the capability to meet the set legal standards for drinking water supply under all circumstances and the operational reliability to solve technical failures without any disturbance of the drinking water supply.
In this research, the resilience of the drinking water supply system is defined as “the possibility to respond to short- and long-term changes in water demand or water quality” (Hashimoto et al., 1982). Climate change and other developments in water demand and quality call for the use of more resilient technologies and processes and may require upgrades of water treatment and storage capacity (WHO and UNICEF, 2017). The cases of “2018 summer drought” and “drinking water demand growth” emphasize the importance of the available abstraction permits and the treatment and distribution capacity compared to the annual and peak water demand, respectively, for the resilience of the local drinking water supply system. Furthermore, the flexibility of the treatment method determines whether a drinking water supply system can deal with variation in, or deterioration of, water quality and emerging contaminants, which are the sustainability aspects found in the case of “groundwater quality development”.
Energy use and environmental impact include the sustainability aspects from the cases of “groundwater quality development” and “drinking water demand growth”. This entails the energy use of abstraction, treatment, and distribution and the environmental impact of additional excipients, wastewater, and other waste products of the treatment. Especially when the raw water quality deteriorates, the required water treatment methods become more complex. In general, this leads to large investments and an increased energy use and environmental impact, e.g. when advanced membrane filtration methods are required. Additional global sustainability aspects are the reliability of the energy supply and the renewability of the energy that is used (WHO, 2017).
3.3 Socio-economic sustainability characteristics
A total of three socio-economic sustainability characteristics are proposed that summarize the socio-economic aspects affecting the drinking water supply as found in the case studies, namely drinking water availability, water governance, and land and water use (Table 3).
Table 3Summary of proposed socio-economic sustainability characteristics, socio-economic aspects from case studies (see Appendices A–C), relevant SDG∗ indicators and WHO Guidelines for Drinking-water Quality (WHO, 2017) aspects, and socio-economic sustainability criteria.
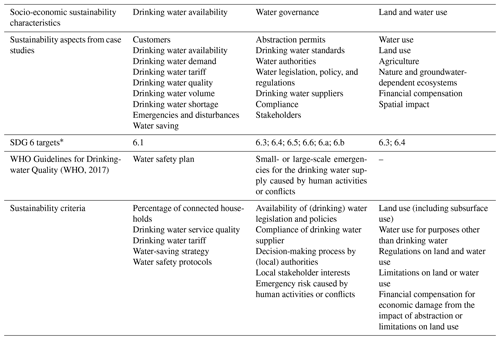
∗ SDG – Sustainable Development Goal; see Appendix V for a summary of SDG 6 targets and indicators related to sustainability characteristics (UN, 2015).
The drinking water availability can be quantified by the percentage of households connected to the drinking water supply. A sustainable local drinking water supply provides sufficient drinking water of a quality that meets the national or international drinking water standards at a tariff that is affordable to all households (UN, 2015). In the Netherlands, by law the drinking water tariff must be built on a cost-recovery, transparent, and non-discriminatory basis (Dutch Government, 2009). Water-saving strategies will reduce the drinking water demand growth and, therefore, will contribute to the sustainability. Drinking water safety is a prerequisite for public health and sustainable drinking water supply. The WHO guidelines consider water safety plans essential for providing the basis for system protection and process control and for ensuring that water quality issues present a negligible risk to public health and that the drinking water is acceptable to consumers. Therefore, the WHO Guidelines for Drinking-water Quality (2017) monitor the availability of water safety plans, including emergency plans on how to act in case of drinking water supply disturbances, shortages, or drinking water quality emergencies (WHO and UNICEF, 2017). A water safety plan can be built on various safety protocols.
Water governance focuses on policies and legislation, enforcement, and compliance of regulations. Good governance also includes decision-making processes that consider different stakeholder interests to ensure accountable, transparent, and participatory governance (UNESCAP, 2009). The availability of (inter-) national and local policies and legislation on drinking water supply as well as on water management, including regulations and permits, and the level of compliance of the drinking water supplier to these policies and legislation, is important for socio-economic sustainability. The sustainability of the local drinking water supply is also characterized by the stakeholders' interests related to the presence of a local drinking water abstraction and by how local authorities weigh these interests in their decision-making processes. A final aspect in water governance that reaches further than local stakeholder interests is the risk of small- or large-scale emergencies for the drinking water supply caused by human activities or conflicts (WHO and UNICEF, 2017).
The local land and water use, at surface and subsurface level, affects the water quality and quantity. It may have resulted in historical contaminant sources, causing point or non-point water pollution, but it may also lead to emerging contaminants that provide new risks to water quality. Additionally, water use for other purposes may limit the availability of water resources for drinking water. Regulations to protect water quality or water quantity may cause limitations on local land and water use. Financial compensation for suffered economic damage due to the impact of the abstraction or the limitations caused by protection regulations can be an important aspect for the sustainability of the drinking water supply system.
4.1 Use of DPSIR systems approach
In this study, we used an integrated systems approach to analyse the local drinking water supply system, combining hydrological, technical, and socio-economic aspects of the system. The analysis of the three selected cases with DPSIR supported the identification of the aspects that shape the sustainability of the local drinking water supply system. The case analysis did indeed help to account for differences between short-term and long-term developments and for the impact of external influences that come from the national and international scale.
The applied DPSIR approach is a linear socio-ecological framework originally developed to identify the impact of human activities on the state of the environmental system (Binder et al., 2013). However, the local drinking water supply system is a complex rather than linear system because the impact of pressure on one system element could lead to pressure on another system element. This complicated the identification of pressures and impacts. For instance, high temperatures and lack of precipitation caused a higher drinking water demand and surface water quality deterioration. Both consequently presented pressures with an impact on the resilience and reliability of the technical drinking water supply infrastructure. Although this hampered the analysis, the use of DPSIR supported a systematic analysis of the local drinking water supply cases and helped to identify the sustainability aspects. Use of a different integrated systems approach would not have led to a significantly different outcome for the case analysis. A next step could potentially be to use the identified system characteristics for system dynamics analysis and modelling. However, this is beyond the scope of this current research.
4.2 General applicability of the sustainability characteristics
To increase the general applicability of the results from the analysis of the Dutch cases on drinking water supply, the identified sustainability aspects were related to worldwide acknowledged sustainability aspects by cross-checking with international policies on drinking water supply. This put the aspects in a broader perspective, which may contribute to the transferability of the proposed sustainability characteristics and criteria to other areas.
Assessments to understand the sustainability challenges and the impact of future developments and adaptation options are seen as powerful tools for policy-making (Ness et al., 2007; Singh et al., 2012). The sustainability characteristics, as proposed in this research, may be used to develop a sustainability assessment for the local drinking water supply system that can help to identify sustainability challenges and trade-offs of adaptation strategies. Trade-off analysis supports decision-making processes and makes these processes more transparent to local stakeholders (Hellegers and Leflaive, 2015). Based on the local situation and data availability, adequate indicators and indices can be selected to quantify the sustainability characteristics in a certain area (Van Engelenburg et al., 2019).
The aim of this study was to identify a set of characteristics that describe the sustainability of a local drinking water supply system in the Netherlands to support policy- and decision-making on sustainable drinking water supply. The use of the DPSIR systems approach was an adequate method for the analysis of the cases. The results of the analysis of the three cases confirmed the hypothesis that sustainability is contextual, resulting in different sustainability aspects in the various cases. The combined results of the analysis of three different practice cases contributed to a better understanding of drinking water supply in the Netherlands. Cross-checking of the results of case analysis with international policies on drinking water supply provided a wider context than the Netherlands and has thus contributed to the general applicability of the identified sustainability characteristics.
Based on the presented analysis, the following set of hydrological, technical, and socio-economic sustainability characteristics is proposed, respectively: (1) water quality, water resource availability, and impact of drinking water abstraction; (2) reliability and resilience of the technical system and energy use and environmental impact; (3) drinking water availability, water governance, and land and water use. An elaboration of the sustainability characteristics into more detailed criteria may further increase the value of the results of this research in the process of the development of policies on sustainable drinking water supply in the Netherlands.
Table A1Summary of the impact, short- and long-term response, and sustainability aspects in case 1 – 2018 summer drought. In the subsequent Table A2, the full results of the case study are presented.
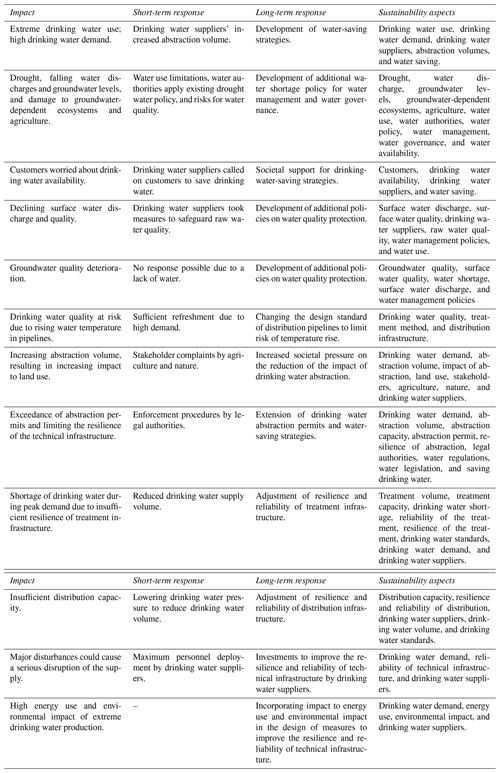
Table C1Summary of the impact, short- and long-term response, and sustainability aspects in case 3 – drinking water demand growth (for complete results of the case study, see Table C2).
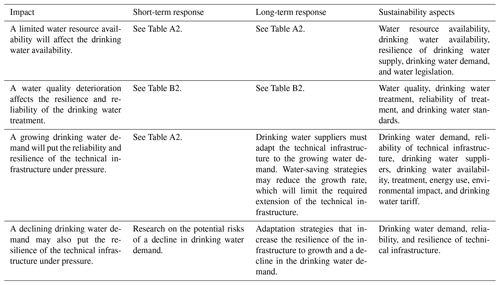
The source data used for the illustrations of the cases are available upon request.
JvE, PH, and EvS conceptualized the study and developed the methodology. JvE curated the data, generated the visualizations, led the investigation, and wrote the original draft. All co-authors reviewed and edited the paper. PH, EvS, AJT, and RU supervised the research (see the CRediT taxonomy for explanations of terms).
The first author performed the research and was partially funded by Vitens, where she is employed. She had carte blanche with respect to the content of the research.
We are indebted to Vitens staff, Maarten Fleuren and Martin de Jonge, for their data collection for the illustrations of the analysed drinking water supply cases, and to Vitens staff, Mark de Vries, Henk Hunneman, and Rian Kloosterman, for cross-checking the results of the case analysis. We thank the two anonymous referees for their comments that helped to further improve the paper.
This paper was edited by Luuk Rietveld and reviewed by two anonymous referees.
Alegre, H., Baptiste, J. M., Cabrera Jr., E., Cubillo, F., Duarte, P., Hirner, W., Merkel, W., and Pareno, R.: Performance Indicators for Water Supply Services, in: Manual of Best Practice, IWA Publishing, London, UK, 2006.
Baggelaar, P. K. and Geudens, P. J. J. G.: Prognoses en scenario's drinkwatergebruik in Nederland (Prognoses and scenarios for drinking water use in the Netherlands), ICASTAT, VEWIN, The Hague, the Netherlands, 2017.
Bauer, J. M. and Herder, P. M.: Designing Socio-Technical Systems. Philosophy of Technology and Engineering Sciences, North-Holland, Amsterdam, the Netherlands, 2009.
Binder, C. R., Hinkel, J., Bots, P. W. G., and Pahl-Wostl, C.: Comparison of frameworks for analyzing social-ecological systems, Ecol. Soc., 18, 26–45, 2013.
Carr, E. R., Wingard, P. M., Yorty, S. C., Thompson, M. C., Jensen, N. K., and Roberson, J.: Applying DPSIR to sustainable development, Int. J Sust. Dev. World, 14, 543–555, 2009.
Cohen, D. A.: The Rationed City: The Politics of Water, Housing, and Land Use in Drought-Parched S ao Paulo, Public Culture, 28, 261–289, 2016.
Dutch Government: Drinkwaterwet (Dutch Drinking water Decree), BWBR0026338, The Hague, the Netherlands, 2009a.
Dutch Government: Waterwet (Dutch Decree on Water), BWBR0025458, The Hague, the Netherlands, 2009b.
Ekins, P., Gupta, J., and Boileau, P.: Global Environment Outlook GEO-6: Healthy Planet, Healthy People. Global Environment Outlook, United Nations Environment Programme, Cambridge, UK, 2019.
European Benchmarking Co-operation: Learning from international best practices; 2017 Water and Wastewater Benchmark, EBC, The Hague, the Netherlands, 2017.
European Environment Agency: European waters Assessment of status and pressures 2018, Luxembourg, 2018.
European Union: EU Water Framework Directive, in: Official Journal of the European Communities, edited by: EUROPEAN UNION, Brussels, Belgium, 2000.
Eurostat: Environmental indicators: Typology and overview, Technical Report N0. 25, European Environment Agency, Copenhagen, Denmark, 1999.
Gleeson, T. and Wada, Y.: Assessing regional groundwater stress for nations using multiple data sources with the groundwater footprint, Environ. Res. Lett., 8, 044010, https://doi.org/10.1088/1748-9326/8/4/044010, 2013.
Hashimoto, T., Stedinger, J. R., and Loucks, D. P.: Reliability, Resiliency, and Vulnerability Criteria For Water Resource System Performance Evaluation, Water Resour. Res., 18, 14–20, 1982.
Hellegers, P. and Leflaive, X.: Water allocation reform: what makes it so difficult?, Water Int., 40, 273–285, 2015.
Janza, M.: A decision support system for emergency response to groundwater resource pollution in an urban area (Ljubljana, Slovenia), Environ. Earth Sci., 73, 3763–3774, 2015.
Jorgensen, L. F. and Stockmarr, J.: Groundwater monitoring in Denmark: Characteristics, perspectives and comparison with other countries, Hydrogeol. J., 17, 827–842, 2009.
Kools, S., Van Loon, A., Sjerps, R., and Rosenthal, L.: The quality of drinking water resources in the Netherlands, edited by: KWR, KWR Report, KWR, Nieuwegein, the Netherlands, 2019.
Liu, J., Mooney, H., Hull, V., Davis, S. J., Gaskell, J., Hertel, T., Lubchenco, J., Seto, K. C., Gleick, P., Kremen, C., and Li, S.: Sustainability. Systems integration for global sustainability, Science, 347, 1258832, https://doi.org/10.1126/science.1258832, 2015.
Loucks, D. P.: Sustainable Water Resources Management, Water Int., 25, 3–10, 2000.
Loucks, D. P., Van Beek, E., Stedinger, J. R., Dijkman, J. P., and Villars, M. T.: Water resource systems planning and management: an introduction to Methods, Models and Applications, Springer, Deltares and UNESCO-IHE, Cham, Switzerland, 2017.
Melese, Y. G., Heijnen, P. W., Stikkelman, R. M., and Herder, P. M.: Exploring for real options during CCS networks conceptual design to mitigate effects of path-dependency and lock-in, Int. J. Greenh. Gas Con., 42, 16–25, 2015.
Mendizabal, I., Baggelaar, P. K., and Stuyfzand, P. J.: Hydrochemical trends for public supply well fields in the Netherlands (1898–2008), natural backgrounds and upscaling to groundwater bodies, J. Hydrol., 450–451, 279–292, 2012.
Ministry of Infrastructure and Environment and Ministry of Economic Affairs and Climate Policy: Nederland beter weerbaar tegen droogte; Eindrapportage Beleidstafel Droogte (the Netherlands more resilient to drought; final report policy table drought), The Hague, the Netherlands, 2019.
Napoli, C. and Garcia-Tellez, B.: A framework for understanding energy for water, Int. J. Water Resour. D, 32, 339–361, 2016.
Ness, B., Urbel-Piirsalu, E., Anderberg, S., and Olsson, L.: Categorising tools for sustainability assessment, Ecol. Econ., 60, 498–508, 2007.
Pahl-Wostl, C.: Towards sustainability in the water sector – The importance of human actors and processes of social learning, Aquat. Sci., 64, 394–411, 2002.
Pahl-Wostl, C.: Water Governance in the Face of Global Change; From understanding to transformation, Springer, London, UK, 2015.
Pant, L. P., Adhikari, B., and Bhattarai, K. K.: Adaptive transition for transformations to sustainability in developing countries, Curr. Opin. Env. Sust., 14, 206–212, 2015.
Singh, R. K., Murty, H. R., Gupta, S. K., and Dikshit, A. K.: An overview of sustainability assessment methodologies, Ecol. Indic., 15, 281–299, 2012.
Smith, A. and Stirling, A.: The Politics of Social-ecological Resilience and Sustainable Socio-technical Transitions, Ecol. Soc., 15, 11, available at: https://www.researchgate.net/publication/45227241_The_Politics_of_Social-ecological_Resilience_and_Sustainable_Socio-technical_Transitions (last access: 18 January 2021), 2010.
Sorensen, P.: The chronic water shortage in Cape Town and survival strategies, Int. J. Environ. Stud., 74, 515–527, 2017.
Teuling, A. J.: A hot future for European droughts, Nat. Clim. Change, 8, 364–365, 2018.
UN: Indicators of Sustainable Development: Guidelines and Methodologies, Economic and Social Affairs, United Nations, New York, USA, 2007.
UN: Transforming our world: The 2030 Agenda for Sustainable Development, United Nations, New York, USA, 2015.
UN: Sustainable Development Goal 6 Synthesis Report 2018 on Water and Sanitation, edited by: Water, U., United Nations, New York, USA, 2018.
UNESCAP: Economic and social survey of Asia and the Pacific 2009; Addressing triple threats to development, edited by: United Nations Economic and Social Commission for Asia and the Pacific, ST/ESCAP/2522, United Nations Economic and Social Commission for Asia and the Pacific, Bangkok, Thailand, 2009.
UNICEF and WHO: Progress on Sanitation and Drinking Water; 2015 Update and MDG Assessment, NLM classification: WA 670, UNICEF and World Health Organization, New York, USA, 2015.
Van den Brink, C. and Wuijts, S.: Towards an effective protection of groundwater resources: putting policy into practice with the drinking water protection file, Water Policy, 18, 635–653, 2016.
Van der Aa, N. G. F. M., Tangena, B. H., Wuijts, S., and De Nijs, A. C. M.: Scenario's drinkwatervraag 2015–2040 en beschikbaarheid bronnen; verkenning grondwatervoorraden voor drinkwater (Scenarios drinking water demand 2015–2040 and water resources availability; exploratory study on groundwater resources for drinking water), RIVM, Bilthoven, the Netherlands, 2015.
Van der Kerk, G. and Manuel, A.: A comprehensive index for a sustainable society: The SSI – the Sustainable Society Index, Ecol. Econ., 66, 228–242, 2008.
Van Engelenburg, J., Hueting, R., Rijpkema, S., Teuling, A. J., Uijlenhoet, R., and Ludwig, F.: Impact of Changes in Groundwater Extractions and Climate Change on Groundwater-Dependent Ecosystems in a Complex Hydrogeological Setting, Water Resour. Manag., 32, 259–272, 2018.
Van Engelenburg, J., Van Slobbe, E., and Hellegers, P.: Towards sustainable drinking water abstraction: an integrated sustainability assessment framework to support local adaptation planning, J. Integr. Environ. Sci., 16, 89–122, 2019.
Van Engelenburg, J., De Jonge, M., Rijpkema, S., Van Slobbe, E., and Bense, V. F.: Hydrogeological evaluation of managed aquifer recharge in a glacial moraine complex using long-term groundwater data analysis, Hydrogeol. J., 28, 1787–1807, 2020.
Van Noordwijk, M., Speelman, E., Hofstede, G. J., Farida, A., Abdurrahim, A. Y., Miccolis, A., Hakim, A. L., Wamucii, C. N., Lagneaux, E., Andreotti, F., Kimbowa, G., Assogba, G. G. C., Best, L., Tanika, L., Githinji, M., Rosero, P., Sari, R. R., Satnarain, U., Adiwibowo, S., Ligtenberg, A., Muthuri, C., Pena-Claros, M., Purwanto, E., Van Oel, P., Rozendaal, D., Suprayogo, D., and Teuling, A. J.: Sustainable Agroforestry Landscape Management: Changing the Game, Land, 9, 243, https://doi.org/10.3390/land9080243, 2020.
Van Thiel, L.: Watergebruik thuis 2016 (Domestic water use 2016). TNO Nipo Report C8732; Kantar Public, Amsterdam, the Netherlands, 2017.
Vitens: Resiliently ahead; Long-term vision on our infrastructure 2016–2040, Vitens, Zwolle, the Netherlands, 2016.
WHO: Guidelines for drinking-water quality: fourth edition incorporating the first addendum, World Health Organization, Geneva, Switzerland, 2017.
WHO and UNICEF: Progress on drinking water, sanitation and hygiene, 2017 update and SDG Baselines, World Health Organization (WHO) and UNICEF, Geneva, Switzerland, 2017.
Wolters, H. A., Van den Born, G. J., Dammers, E., and Reinhard, S.: Deltascenario's voor de 21e eeuw, actualisering 2017 (Delta scenarios for the 21st century, actualisation 2017), Deltares, Utrecht, the Netherlands, 2018.
Raw water is the (untreated) water that is treated to produce drinking water. This can be abstracted groundwater or surface water, depending on the available water resource.
- Abstract
- Introduction
- Method
- Sustainability characteristics of drinking water supply
- Discussion
- Conclusions
- Appendix A: Results of analysis case 1: 2018 summer drought
- Appendix B: Results of analysis case 2: groundwater quality development
- Appendix C: Results of analysis case 3: drinking water demand growth
- Appendix D: Summary of Sustainable Development Goal 6 targets and indicators related to sustainability characteristics
- Appendix E: Overview of sustainability characteristics and criteria
- Data availability
- Author contributions
- Competing interests
- Acknowledgements
- Review statement
- References
- Abstract
- Introduction
- Method
- Sustainability characteristics of drinking water supply
- Discussion
- Conclusions
- Appendix A: Results of analysis case 1: 2018 summer drought
- Appendix B: Results of analysis case 2: groundwater quality development
- Appendix C: Results of analysis case 3: drinking water demand growth
- Appendix D: Summary of Sustainable Development Goal 6 targets and indicators related to sustainability characteristics
- Appendix E: Overview of sustainability characteristics and criteria
- Data availability
- Author contributions
- Competing interests
- Acknowledgements
- Review statement
- References